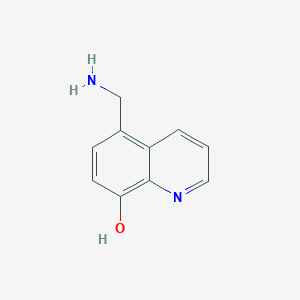
5-(Aminomethyl)quinolin-8-ol
Vue d'ensemble
Description
5-(Aminomethyl)quinolin-8-ol (CAS No. 13207-66-4) is an 8-hydroxyquinoline derivative featuring an aminomethyl (-CH$2$NH$2$) substituent at the C5 position of the quinoline core. Its molecular formula is C$9$H$8$N$_2$O, with a molecular weight of 160.17 g/mol. This compound serves as a versatile intermediate in medicinal chemistry due to its ability to undergo further functionalization, such as alkylation, acylation, or conjugation with metal ions . The primary amine group at C5 enhances its chelation properties, making it relevant in corrosion inhibition and metal-binding applications .
Méthodes De Préparation
Synthetic Routes and Reaction Conditions
The synthesis of 5-(Aminomethyl)quinolin-8-ol typically involves the reaction of 5-(chloromethyl)quinolin-8-ol with hexamethylenetetramine in dimethyl sulfoxide at room temperature. This is followed by treatment with hydrochloric acid in water at room temperature for 48 hours .
Industrial Production Methods
While specific industrial production methods for this compound are not widely documented, the general approach involves large-scale synthesis using similar reaction conditions as in laboratory settings, with optimizations for yield and purity.
Analyse Des Réactions Chimiques
Types of Reactions
5-(Aminomethyl)quinolin-8-ol undergoes various chemical reactions, including:
Oxidation: This reaction involves the addition of oxygen or the removal of hydrogen, often using oxidizing agents.
Reduction: This reaction involves the addition of hydrogen or the removal of oxygen, typically using reducing agents.
Substitution: This reaction involves the replacement of one functional group with another, often using nucleophiles or electrophiles.
Common Reagents and Conditions
Oxidation: Common oxidizing agents include potassium permanganate and hydrogen peroxide.
Reduction: Common reducing agents include sodium borohydride and lithium aluminum hydride.
Substitution: Common reagents include halogens, acids, and bases.
Major Products Formed
The major products formed from these reactions depend on the specific reagents and conditions used. For example, oxidation may yield quinolin-8-ol derivatives, while reduction may yield various aminomethylated products.
Applications De Recherche Scientifique
Anticancer Properties
5-(Aminomethyl)quinolin-8-ol exhibits significant anticancer activity, particularly through its ability to inhibit proteasome activity. Studies have shown that this compound can enhance the efficacy of existing chemotherapeutic agents such as bortezomib, which is used to treat multiple myeloma. The compound's interaction with bovine serum albumin suggests a favorable pharmacokinetic profile, enhancing its potential as an oral medication due to high water solubility and intestinal absorptivity .
Case Study: Interaction with Serum Proteins
In silico and multi-spectroscopic analyses demonstrated that this compound binds effectively to serum proteins, indicating a potential for improved drug delivery mechanisms. The binding occurs at specific sites within the protein structure, which could facilitate targeted therapy in cancer treatment .
Metal Ion Chelation
The compound's chelating properties are notable, particularly in the context of neurodegenerative diseases where metal ion imbalance plays a critical role. This compound can chelate metal ions such as copper and iron, which are implicated in oxidative stress and neurodegeneration . This property is essential for developing treatments aimed at restoring metal homeostasis in patients with conditions like Alzheimer’s disease and Parkinson’s disease.
Table 1: Chelation Properties of this compound
Metal Ion | Chelation Efficacy | Biological Relevance |
---|---|---|
Copper | High | Involved in oxidative stress |
Iron | Moderate | Linked to neurodegeneration |
Zinc | Low | Non-redox reactive |
Material Science Applications
Recent advancements have seen the incorporation of this compound into electrospun materials for biomedical applications. These materials demonstrate antibacterial and antifungal properties alongside their anticancer effects. For instance, electrospun fibers containing this compound have shown significant cytotoxicity against cancer cell lines such as HeLa and MCF-7 while exhibiting lower toxicity towards normal cells . This dual functionality makes these materials suitable candidates for drug delivery systems and wound healing applications.
Case Study: Electrospun Fibers
Electrospinning techniques have been employed to create fibrous materials that incorporate this compound. These fibers not only deliver the compound effectively but also enhance its biological activity through metal ion complexation, improving therapeutic outcomes in local cancer therapies .
Structure-Activity Relationship (SAR)
Understanding the structure-activity relationship of this compound is crucial for optimizing its therapeutic potential. Modifications at various positions on the quinoline ring can significantly influence its bioactivity and selectivity towards cancer cells or specific metal ions.
Table 2: Structure-Activity Relationship Insights
Modification Position | Effect on Activity | Notes |
---|---|---|
C6 or C7 | Increased NQO1 binding affinity | Enhances cytotoxicity |
Amino Group | Improved solubility and binding to proteins | Facilitates oral administration |
Mécanisme D'action
The mechanism of action of 5-(Aminomethyl)quinolin-8-ol involves its interaction with molecular targets such as enzymes and receptors. It can inhibit the activity of certain enzymes, leading to the disruption of biological pathways. For example, quinoline derivatives are known to inhibit DNA synthesis by promoting the cleavage of bacterial DNA gyrase and type IV topoisomerase, ultimately leading to rapid bacterial death .
Comparaison Avec Des Composés Similaires
Comparison with Structural Analogues
Antiviral Derivatives
- 5-((p-Tolylamino)methyl)quinolin-8-ol and 5-(((3,4-dimethylphenyl)amino)methyl)quinolin-8-ol (): Substituent: Aromatic amino groups (-CH$2$NHAr). Activity: Inhibitors of the HIV-1 Integrase-LEDGF/p75 interaction with low micromolar EC${50}$ values (antiviral activity). Key Findings: These derivatives exhibit potent antiviral effects while maintaining low cytotoxicity, attributed to the bulky aryl groups enhancing target binding . Comparison: Unlike the primary amine in 5-(Aminomethyl)quinolin-8-ol, the aromatic amines in these analogues improve hydrophobic interactions with the target protein, increasing potency.
Corrosion Inhibitors
- 5-(Azidomethyl)quinolin-8-ol (8QN3) (): Substituent: Azidomethyl (-CH$2$N$3$). Activity: Corrosion inhibition for mild steel in HCl (90% efficiency at 5×10$^{-3}$ M). Key Findings: The azide group enhances adsorption on metal surfaces via electron-rich interactions, outperforming benzylamino and acetamide derivatives . Comparison: The aminomethyl group in this compound may offer weaker adsorption due to its lower electron-withdrawing capacity compared to the azide.
Antibacterial Agents
- 5-((4-Methylpiperazin-1-yl)methyl)quinolin-8-ol (): Substituent: Piperazine (-CH$_2$-piperazine). Activity: Antibacterial activity against Gram-positive bacteria (e.g., Staphylococcus aureus). Key Findings: The piperazine moiety improves solubility and bacterial membrane penetration, critical for antimicrobial efficacy . Comparison: The simpler aminomethyl group lacks the steric bulk and hydrogen-bonding capacity of piperazine, limiting direct antibacterial action without further derivatization.
Enzyme Inhibitors
- 5-(Morpholin-4-ylmethyl)quinolin-8-ol (): Substituent: Morpholine (-CH$_2$-morpholine). Activity: Inhibition of UGGT (ER glycoprotein folding enzyme) with a co-crystal structure resolved at 1.65 Å. Key Findings: The morpholine ring facilitates hydrogen bonding and van der Waals interactions within the enzyme active site . Comparison: The primary amine in this compound lacks the cyclic ether oxygen of morpholine, reducing its fit in UGGT’s binding pocket.
Activité Biologique
5-(Aminomethyl)quinolin-8-ol, also known as 5-aminomethyl-8-hydroxyquinoline, is a compound that has garnered attention in medicinal chemistry due to its diverse biological activities. This article explores the synthesis, biological properties, mechanisms of action, and potential applications of this compound based on recent research findings.
Chemical Structure and Properties
This compound is characterized by its quinoline backbone with an amino group at the 5-position and a hydroxyl group at the 8-position. The molecular formula is , and its molecular weight is approximately 174.20 g/mol.
Property | Value |
---|---|
Molecular Formula | |
Molecular Weight | 174.20 g/mol |
CAS Number | 81748-72-3 |
Synthesis Methods
The synthesis of this compound typically involves the Mannich reaction, where 8-hydroxyquinoline is reacted with formaldehyde and an amine. This method allows for the introduction of the aminomethyl group at the desired position on the quinoline ring, yielding the target compound in good yields.
Antimicrobial Properties
Research has indicated that this compound exhibits significant antimicrobial activity against various bacterial strains. For instance, derivatives of this compound have shown bactericidal effects against Gram-positive bacteria such as Staphylococcus aureus and Bacillus subtilis . The Minimum Inhibitory Concentration (MIC) values for these compounds range from to M, demonstrating their potential as effective antimicrobial agents.
Anticancer Activity
Several studies have highlighted the anticancer properties of this compound derivatives. These compounds have been shown to inhibit cell proliferation in various cancer cell lines, including breast cancer (MCF-7) and colon cancer (HCT116). The mechanism involves the induction of apoptosis and cell cycle arrest through modulation of key signaling pathways such as p53 .
Case Study:
In a study examining the cytotoxic effects of these derivatives, it was found that introducing different substituents at the 5-position significantly influenced their anticancer activity. Compounds with halogen substitutions exhibited enhanced potency compared to unsubstituted analogs .
The biological activity of this compound is attributed to its ability to interact with specific molecular targets within cells. The hydroxyl group can form hydrogen bonds with biological macromolecules, enhancing its binding affinity to enzymes involved in metabolic pathways. Additionally, the amino group may facilitate interactions with nucleophilic sites on proteins or nucleic acids, leading to inhibition of enzyme activity or disruption of cellular processes .
Comparative Analysis with Related Compounds
This compound can be compared to other quinoline derivatives regarding their biological activities:
Compound | Antimicrobial Activity | Anticancer Activity | Mechanism of Action |
---|---|---|---|
This compound | High | Moderate | Enzyme inhibition |
8-Hydroxyquinoline | Moderate | Low | Metal chelation |
5-Chloroquinolin | High | High | DNA intercalation |
Q & A
Q. Basic: What are the optimal synthetic routes for 5-(Aminomethyl)quinolin-8-ol, and how can purity be ensured?
Answer:
The synthesis typically involves multi-step reactions starting with 8-hydroxyquinoline derivatives. For example, formaldehyde and amines are used to introduce the aminomethyl group via Mannich-type reactions . Key steps include:
- Reagent selection: Paraformaldehyde and amines (e.g., piperidine, morpholine) under reflux in polar solvents like ethanol.
- Purification: Column chromatography (silica gel, eluent: CH₂Cl₂/MeOH) followed by recrystallization to achieve >95% purity .
- Validation: ¹H NMR (e.g., δ 5.31 ppm for methylene protons) and HRMS (e.g., [M+H]⁺ at m/z 175.0974) confirm structure and purity .
Q. Basic: Which spectroscopic techniques are most effective for characterizing this compound derivatives?
Answer:
A combination of techniques is required:
- ¹H/¹³C NMR: Assigns proton environments (e.g., aromatic protons at δ 7.2–8.8 ppm) and carbon backbone .
- UV-Vis: Identifies π→π* transitions (e.g., λₘₐₓ ~400–420 nm for azo derivatives) .
- X-ray crystallography: Resolves 3D structures (e.g., bond angles and dihedral angles for stability analysis) .
- FT-IR: Confirms functional groups (e.g., O–H stretch at ~3200 cm⁻¹) .
Q. Advanced: How can computational modeling guide the design of this compound-based enzyme inhibitors?
Answer:
- Molecular docking: Predict binding affinity to targets like KDM3B (IC₅₀ <10 µM) or HIV-1 integrase . Tools like AutoDock Vina assess interactions (e.g., hydrogen bonds with catalytic residues).
- DFT calculations: Optimize geometry and electron distribution (e.g., HOMO-LUMO gaps for redox activity) .
- Validation: Compare computational results with X-ray crystallography (e.g., RMSD <1.5 Å) and bioassays .
Q. Advanced: How do substituent modifications influence the biological activity of this compound derivatives?
Answer:
- Structure-Activity Relationship (SAR):
- Testing: Use in vitro assays (e.g., viral replication inhibition in MT-4 cells) and correlate with logD/ClogP values .
Q. Advanced: How can contradictory data in corrosion inhibition studies be resolved?
Answer:
- Systematic review: Follow PRISMA guidelines to screen literature (e.g., 2 independent reviewers for data extraction) .
- Experimental validation: Compare electrochemical impedance spectroscopy (EIS) and weight loss methods under standardized conditions (e.g., 1 M HCl, 25°C) .
- Computational cross-check: Use DFT to model adsorption energies (e.g., ΔGₐdₛ <−30 kJ/mol for effective inhibitors) .
Q. Advanced: What methodologies elucidate the corrosion inhibition mechanism of this compound derivatives?
Answer:
- Electrochemical techniques: Potentiodynamic polarization (Tafel slopes) and EIS (charge-transfer resistance) quantify efficiency (>90% at 10⁻³ M) .
- Surface analysis: SEM/EDX confirms inhibitor adsorption on carbon steel .
- Theoretical modeling: Monte Carlo simulations predict adsorption sites on Fe(110) surfaces .
Q. Advanced: How does crystallography aid in understanding the interaction of this compound with biological targets?
Answer:
- Co-crystallization: Soak ligands into protein crystals (e.g., KDM3B at 2.1 Å resolution) to identify binding modes .
- Structural analysis: Measure bond distances (e.g., 2.8 Å between ligand O and Zn²⁺ in metalloenzymes) .
- Fragment-based design: Use crystal structures to optimize substituents (e.g., tetrazole for π-stacking with His residues) .
Q. Advanced: What strategies improve the solubility and bioavailability of this compound derivatives?
Answer:
- Salt formation: Hydrochloride salts (e.g., this compound·HCl) enhance aqueous solubility (>50 mg/mL) .
- Prodrug design: Acetylate hydroxyl groups for passive diffusion, with enzymatic cleavage in vivo .
- Nanoformulation: Encapsulate in liposomes (PDI <0.2) for sustained release .
Q. Advanced: How can Hirshfeld surface analysis refine the supramolecular properties of this compound complexes?
Answer:
- Crystal structure input: Generate surfaces using CrystalExplorer to quantify intermolecular interactions (e.g., H-bonding vs. van der Waals) .
- Fingerprint plots: Compare dᵢ + dₑ distances (e.g., 1.7–2.8 Å for O–H⋯N contacts) .
- Thermal analysis: Correlate packing motifs with DSC/TGA stability data .
Q. Advanced: What are the best practices for synthesizing azo derivatives of this compound?
Answer:
Propriétés
IUPAC Name |
5-(aminomethyl)quinolin-8-ol | |
---|---|---|
Source | PubChem | |
URL | https://pubchem.ncbi.nlm.nih.gov | |
Description | Data deposited in or computed by PubChem | |
InChI |
InChI=1S/C10H10N2O/c11-6-7-3-4-9(13)10-8(7)2-1-5-12-10/h1-5,13H,6,11H2 | |
Source | PubChem | |
URL | https://pubchem.ncbi.nlm.nih.gov | |
Description | Data deposited in or computed by PubChem | |
InChI Key |
KMNQWCOAYXOQKY-UHFFFAOYSA-N | |
Source | PubChem | |
URL | https://pubchem.ncbi.nlm.nih.gov | |
Description | Data deposited in or computed by PubChem | |
Canonical SMILES |
C1=CC2=C(C=CC(=C2N=C1)O)CN | |
Source | PubChem | |
URL | https://pubchem.ncbi.nlm.nih.gov | |
Description | Data deposited in or computed by PubChem | |
Molecular Formula |
C10H10N2O | |
Source | PubChem | |
URL | https://pubchem.ncbi.nlm.nih.gov | |
Description | Data deposited in or computed by PubChem | |
DSSTOX Substance ID |
DTXSID80629733 | |
Record name | 5-(Aminomethyl)quinolin-8-ol | |
Source | EPA DSSTox | |
URL | https://comptox.epa.gov/dashboard/DTXSID80629733 | |
Description | DSSTox provides a high quality public chemistry resource for supporting improved predictive toxicology. | |
Molecular Weight |
174.20 g/mol | |
Source | PubChem | |
URL | https://pubchem.ncbi.nlm.nih.gov | |
Description | Data deposited in or computed by PubChem | |
CAS No. |
81748-72-3 | |
Record name | 5-(Aminomethyl)quinolin-8-ol | |
Source | EPA DSSTox | |
URL | https://comptox.epa.gov/dashboard/DTXSID80629733 | |
Description | DSSTox provides a high quality public chemistry resource for supporting improved predictive toxicology. | |
Retrosynthesis Analysis
AI-Powered Synthesis Planning: Our tool employs the Template_relevance Pistachio, Template_relevance Bkms_metabolic, Template_relevance Pistachio_ringbreaker, Template_relevance Reaxys, Template_relevance Reaxys_biocatalysis model, leveraging a vast database of chemical reactions to predict feasible synthetic routes.
One-Step Synthesis Focus: Specifically designed for one-step synthesis, it provides concise and direct routes for your target compounds, streamlining the synthesis process.
Accurate Predictions: Utilizing the extensive PISTACHIO, BKMS_METABOLIC, PISTACHIO_RINGBREAKER, REAXYS, REAXYS_BIOCATALYSIS database, our tool offers high-accuracy predictions, reflecting the latest in chemical research and data.
Strategy Settings
Precursor scoring | Relevance Heuristic |
---|---|
Min. plausibility | 0.01 |
Model | Template_relevance |
Template Set | Pistachio/Bkms_metabolic/Pistachio_ringbreaker/Reaxys/Reaxys_biocatalysis |
Top-N result to add to graph | 6 |
Feasible Synthetic Routes
Avertissement et informations sur les produits de recherche in vitro
Veuillez noter que tous les articles et informations sur les produits présentés sur BenchChem sont destinés uniquement à des fins informatives. Les produits disponibles à l'achat sur BenchChem sont spécifiquement conçus pour des études in vitro, qui sont réalisées en dehors des organismes vivants. Les études in vitro, dérivées du terme latin "in verre", impliquent des expériences réalisées dans des environnements de laboratoire contrôlés à l'aide de cellules ou de tissus. Il est important de noter que ces produits ne sont pas classés comme médicaments et n'ont pas reçu l'approbation de la FDA pour la prévention, le traitement ou la guérison de toute condition médicale, affection ou maladie. Nous devons souligner que toute forme d'introduction corporelle de ces produits chez les humains ou les animaux est strictement interdite par la loi. Il est essentiel de respecter ces directives pour assurer la conformité aux normes légales et éthiques en matière de recherche et d'expérimentation.