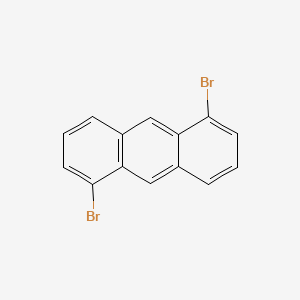
1,5-Dibromoanthracene
Vue d'ensemble
Description
1,5-Dibromoanthracene is a derivative of anthracene, a polycyclic aromatic hydrocarbon. This compound is characterized by the presence of two bromine atoms attached to the 1 and 5 positions of the anthracene ring system. The molecular formula of this compound is C₁₄H₈Br₂, and it has a molecular weight of 336.03 g/mol . This compound is known for its unique photophysical properties and is used in various scientific research applications.
Mécanisme D'action
Target of Action
1,5-Dibromoanthracene is a derivative of anthracene with two bromine atoms
Mode of Action
It’s known that anthracene derivatives can participate in [4+2] annulation reactions . This reaction involves wavelength-selective agitation of 4CzIPN, energy transfer to quinones, recombination of 1,6-biradicals, and elimination to give anthracenone-furans .
Pharmacokinetics
The physicochemical properties of the compound, such as its molecular weight (33603 g/mol ) and melting point (209 °C ), can influence its bioavailability.
Result of Action
Anthracene derivatives are known to exhibit various bioactivities, such as antimicrobial, antiviral, anticancer, and anti-inflammatory activities .
Action Environment
The action of this compound can be influenced by various environmental factors. For instance, the position of the substituents on the linear anthracene parent molecule significantly impacts intermolecular forces, such as hydrogen bonding and steric repulsion . Additionally, the electronegativity/polarity of a given atom strongly influences the observed fusion behavior .
Analyse Biochimique
Biochemical Properties
1,5-Dibromoanthracene plays a significant role in biochemical reactions, particularly in the context of proteomics research. It interacts with various enzymes, proteins, and other biomolecules. The compound’s bromine atoms can participate in halogen bonding, which can influence the binding affinity and specificity of enzymes and proteins. For instance, this compound has been shown to interact with cytochrome P450 enzymes, affecting their catalytic activity and substrate specificity . Additionally, it can form complexes with proteins through non-covalent interactions, impacting protein folding and stability.
Cellular Effects
The effects of this compound on cellular processes are multifaceted. It has been observed to influence cell signaling pathways, gene expression, and cellular metabolism. In particular, this compound can modulate the activity of kinases and phosphatases, leading to alterations in phosphorylation states of key signaling proteins. This modulation can affect downstream signaling pathways, such as the MAPK and PI3K/Akt pathways, ultimately influencing cell proliferation, differentiation, and apoptosis . Furthermore, this compound has been reported to impact gene expression by interacting with transcription factors and altering their binding to DNA, thereby regulating the transcription of target genes.
Molecular Mechanism
At the molecular level, this compound exerts its effects through various mechanisms. One key mechanism involves its binding interactions with biomolecules. The bromine atoms in this compound can form halogen bonds with nucleophilic sites on proteins and enzymes, leading to changes in their conformation and activity. Additionally, this compound can act as an inhibitor or activator of enzymes by binding to their active sites or allosteric sites, respectively . This binding can result in either the inhibition or enhancement of enzymatic activity, depending on the nature of the interaction. Moreover, this compound can influence gene expression by interacting with transcriptional regulators and modulating their activity.
Temporal Effects in Laboratory Settings
The stability and degradation of this compound in laboratory settings are crucial factors that influence its effects over time. Studies have shown that this compound is relatively stable under standard laboratory conditions, with minimal degradation observed over extended periods . Its stability can be affected by factors such as temperature, pH, and exposure to light. Long-term studies have indicated that this compound can have sustained effects on cellular function, including alterations in cell proliferation and differentiation, even after prolonged exposure.
Dosage Effects in Animal Models
The effects of this compound vary with different dosages in animal models. At low doses, this compound has been shown to have minimal toxic effects and can modulate specific biochemical pathways without causing significant adverse effects . At higher doses, this compound can induce toxicity, leading to adverse effects such as hepatotoxicity, nephrotoxicity, and oxidative stress. These toxic effects are dose-dependent and highlight the importance of determining the appropriate dosage for therapeutic applications.
Metabolic Pathways
This compound is involved in various metabolic pathways, interacting with enzymes and cofactors that facilitate its metabolism. The compound undergoes phase I and phase II metabolic reactions, including oxidation, reduction, and conjugation . Cytochrome P450 enzymes play a crucial role in the oxidative metabolism of this compound, converting it into more hydrophilic metabolites that can be readily excreted. Additionally, conjugation reactions with glutathione, glucuronic acid, and sulfate further enhance the compound’s solubility and facilitate its elimination from the body.
Transport and Distribution
The transport and distribution of this compound within cells and tissues are mediated by various transporters and binding proteins. The compound can be taken up by cells through passive diffusion and active transport mechanisms . Once inside the cell, this compound can bind to intracellular proteins, influencing its localization and accumulation. The distribution of this compound within tissues is influenced by factors such as tissue perfusion, binding affinity to plasma proteins, and the presence of specific transporters.
Subcellular Localization
The subcellular localization of this compound is critical for its activity and function. The compound can localize to various cellular compartments, including the cytoplasm, nucleus, and mitochondria . Targeting signals and post-translational modifications play a role in directing this compound to specific subcellular compartments. For instance, the presence of nuclear localization signals can facilitate its transport into the nucleus, where it can interact with transcription factors and influence gene expression. Similarly, mitochondrial targeting signals can direct this compound to the mitochondria, affecting mitochondrial function and metabolism.
Méthodes De Préparation
Synthetic Routes and Reaction Conditions
1,5-Dibromoanthracene can be synthesized through the bromination of anthracene. One common method involves the use of bromine (Br₂) in the presence of a solvent such as carbon tetrachloride (CCl₄). The reaction is typically carried out at room temperature, and the product is purified through recrystallization .
Industrial Production Methods
In industrial settings, the production of this compound may involve more efficient and scalable methods. These methods often utilize automated systems for precise control of reaction conditions, ensuring high yield and purity of the final product. The use of advanced purification techniques, such as column chromatography, is also common in industrial production .
Analyse Des Réactions Chimiques
Types of Reactions
1,5-Dibromoanthracene undergoes various chemical reactions, including:
Substitution Reactions: The bromine atoms in this compound can be replaced by other substituents through nucleophilic substitution reactions.
Oxidation Reactions: This compound can be oxidized to form anthraquinone derivatives.
Reduction Reactions: Reduction of this compound can lead to the formation of dihydroanthracene derivatives.
Major Products
The major products formed from these reactions depend on the specific reagents and conditions used. For example, nucleophilic substitution reactions can yield a variety of substituted anthracene derivatives, while oxidation reactions typically produce anthraquinone derivatives .
Applications De Recherche Scientifique
1,5-Dibromoanthracene has several applications in scientific research, including:
Organic Light Emitting Diodes (OLEDs): This compound is used as a building block for the synthesis of blue-emitting materials in OLEDs.
Fluorescent Probes: Due to its photophysical properties, this compound is used in the development of fluorescent probes for biological imaging.
Photon Upconversion: This compound is utilized in triplet–triplet annihilation photon upconversion systems, which are used to convert low-energy photons into higher-energy photons.
Material Science: This compound is used in the synthesis of organic semiconductors and other advanced materials.
Comparaison Avec Des Composés Similaires
1,5-Dibromoanthracene can be compared with other dibromoanthracene isomers, such as:
- 1,2-Dibromoanthracene
- 1,3-Dibromoanthracene
- 1,4-Dibromoanthracene
- 1,6-Dibromoanthracene
- 1,8-Dibromoanthracene
- 1,9-Dibromoanthracene
- 1,10-Dibromoanthracene
- 2,3-Dibromoanthracene
- 2,6-Dibromoanthracene
- 2,7-Dibromoanthracene
- 3,9-Dibromoanthracene
- 9,10-Dibromoanthracene
Each of these isomers has unique properties and applications, depending on the position of the bromine atoms on the anthracene ring. For example, 9,10-Dibromoanthracene is commonly used in the synthesis of organic semiconductors and has different photophysical properties compared to this compound .
Propriétés
IUPAC Name |
1,5-dibromoanthracene | |
---|---|---|
Source | PubChem | |
URL | https://pubchem.ncbi.nlm.nih.gov | |
Description | Data deposited in or computed by PubChem | |
InChI |
InChI=1S/C14H8Br2/c15-13-5-1-3-9-7-12-10(8-11(9)13)4-2-6-14(12)16/h1-8H | |
Source | PubChem | |
URL | https://pubchem.ncbi.nlm.nih.gov | |
Description | Data deposited in or computed by PubChem | |
InChI Key |
DIMYVOCPPKNNPF-UHFFFAOYSA-N | |
Source | PubChem | |
URL | https://pubchem.ncbi.nlm.nih.gov | |
Description | Data deposited in or computed by PubChem | |
Canonical SMILES |
C1=CC2=CC3=C(C=CC=C3Br)C=C2C(=C1)Br | |
Source | PubChem | |
URL | https://pubchem.ncbi.nlm.nih.gov | |
Description | Data deposited in or computed by PubChem | |
Molecular Formula |
C14H8Br2 | |
Source | PubChem | |
URL | https://pubchem.ncbi.nlm.nih.gov | |
Description | Data deposited in or computed by PubChem | |
DSSTOX Substance ID |
DTXSID30565495 | |
Record name | 1,5-Dibromoanthracene | |
Source | EPA DSSTox | |
URL | https://comptox.epa.gov/dashboard/DTXSID30565495 | |
Description | DSSTox provides a high quality public chemistry resource for supporting improved predictive toxicology. | |
Molecular Weight |
336.02 g/mol | |
Source | PubChem | |
URL | https://pubchem.ncbi.nlm.nih.gov | |
Description | Data deposited in or computed by PubChem | |
CAS No. |
3278-82-8 | |
Record name | 1,5-Dibromoanthracene | |
Source | EPA DSSTox | |
URL | https://comptox.epa.gov/dashboard/DTXSID30565495 | |
Description | DSSTox provides a high quality public chemistry resource for supporting improved predictive toxicology. | |
Retrosynthesis Analysis
AI-Powered Synthesis Planning: Our tool employs the Template_relevance Pistachio, Template_relevance Bkms_metabolic, Template_relevance Pistachio_ringbreaker, Template_relevance Reaxys, Template_relevance Reaxys_biocatalysis model, leveraging a vast database of chemical reactions to predict feasible synthetic routes.
One-Step Synthesis Focus: Specifically designed for one-step synthesis, it provides concise and direct routes for your target compounds, streamlining the synthesis process.
Accurate Predictions: Utilizing the extensive PISTACHIO, BKMS_METABOLIC, PISTACHIO_RINGBREAKER, REAXYS, REAXYS_BIOCATALYSIS database, our tool offers high-accuracy predictions, reflecting the latest in chemical research and data.
Strategy Settings
Precursor scoring | Relevance Heuristic |
---|---|
Min. plausibility | 0.01 |
Model | Template_relevance |
Template Set | Pistachio/Bkms_metabolic/Pistachio_ringbreaker/Reaxys/Reaxys_biocatalysis |
Top-N result to add to graph | 6 |
Feasible Synthetic Routes
Q1: What makes 1,5-Dibromoanthracene a useful starting material in organic synthesis?
A1: this compound is a valuable building block for creating complex polycyclic aromatic hydrocarbons (PAHs). Its structure, with bromine atoms at the 1 and 5 positions, allows for selective modification and the introduction of various functional groups. [, ] For example, it can be reacted with butyllithium to generate a dilithio derivative, which then reacts with electrophiles to yield diversely substituted anthracene derivatives.
Q2: Can you give an example of how this compound is used to synthesize complex molecules with interesting properties?
A2: Certainly! In one study [], researchers used this compound to synthesize elongated polycyclic arenes with specific carboxylic acid substitution patterns. These resulting molecules exhibited interesting optical properties, particularly their absorption and emission spectra, which are crucial for applications in optoelectronics and materials science. They achieved this by first reacting this compound with butyllithium and diethyloxalate, followed by saponification to yield anthrylene-1,5-diglyoxylic acid. This bifunctional molecule was then used in a double condensation reaction with either 2-bromophenylacetic acid or 1-naphthylglyoxylic acid, followed by cyclization, to produce two different isomeric diimides. The striking difference in the colors of these isomers (bright orange vs. red) highlighted the impact of substituent position on the electronic properties of the final molecules.
Q3: Are there other ways to utilize the reactivity of this compound in synthesis?
A3: Yes, this compound can undergo enantioselective alkynylation reactions. [] This method allows for the controlled introduction of chiral alkyne groups, opening up possibilities for the synthesis of chiral triptycene derivatives, which are important in fields like asymmetric catalysis and materials science.
Avertissement et informations sur les produits de recherche in vitro
Veuillez noter que tous les articles et informations sur les produits présentés sur BenchChem sont destinés uniquement à des fins informatives. Les produits disponibles à l'achat sur BenchChem sont spécifiquement conçus pour des études in vitro, qui sont réalisées en dehors des organismes vivants. Les études in vitro, dérivées du terme latin "in verre", impliquent des expériences réalisées dans des environnements de laboratoire contrôlés à l'aide de cellules ou de tissus. Il est important de noter que ces produits ne sont pas classés comme médicaments et n'ont pas reçu l'approbation de la FDA pour la prévention, le traitement ou la guérison de toute condition médicale, affection ou maladie. Nous devons souligner que toute forme d'introduction corporelle de ces produits chez les humains ou les animaux est strictement interdite par la loi. Il est essentiel de respecter ces directives pour assurer la conformité aux normes légales et éthiques en matière de recherche et d'expérimentation.