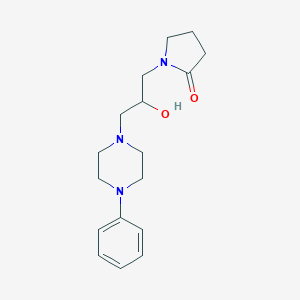
MG 1
Vue d'ensemble
Description
MG 1 is a compound known for its significant pharmacological activities, particularly in the field of cardiovascular medicine. This compound has been studied for its antiarrhythmic and hypotensive properties, making it a potential candidate for therapeutic applications .
Méthodes De Préparation
The synthesis of MG 1 involves several steps. One common synthetic route includes the reaction of 4-phenyl-1-piperazine with 3-chloropropanol to form an intermediate, which is then reacted with 2-pyrrolidinone under specific conditions to yield the final product . Industrial production methods may involve optimization of reaction conditions such as temperature, pressure, and the use of catalysts to enhance yield and purity.
Analyse Des Réactions Chimiques
MG 1 undergoes various chemical reactions, including:
Oxidation: This compound can be oxidized using common oxidizing agents such as potassium permanganate or hydrogen peroxide.
Reduction: Reduction reactions can be carried out using agents like sodium borohydride or lithium aluminum hydride.
Substitution: Nucleophilic substitution reactions can occur, particularly at the hydroxyl group, using reagents like alkyl halides or acyl chlorides.
The major products formed from these reactions depend on the specific reagents and conditions used .
Applications De Recherche Scientifique
MG 1 has been extensively studied for its pharmacological effects. It has shown promise in:
Cardiovascular Medicine: The compound exhibits antiarrhythmic and hypotensive activities, making it a potential therapeutic agent for managing arrhythmias and hypertension.
Biological Research: It is used in studies related to adrenergic receptor antagonism, providing insights into the mechanisms of cardiovascular diseases.
Mécanisme D'action
The mechanism of action of MG 1 involves its interaction with adrenergic receptors. The compound acts as an alpha-adrenoceptor antagonist, which leads to a decrease in systolic and diastolic blood pressure and exhibits antiarrhythmic effects . The S-enantiomer of the compound has been found to be more potent in exerting these effects compared to the R-enantiomer .
Comparaison Avec Des Composés Similaires
MG 1 can be compared with other alpha-adrenoceptor antagonists such as:
Prazosin: Known for its use in treating hypertension and heart failure.
Phentolamine: Used in the management of pheochromocytoma and hypertensive emergencies.
Nicergoline: Utilized for its vasodilatory properties in cerebrovascular disorders.
The uniqueness of this compound lies in its specific structural features and the pronounced pharmacological effects of its S-enantiomer .
Activité Biologique
Magnesium comenate (MG 1), a coordination compound formed from magnesium ions and comenic acid, has garnered attention for its diverse biological activities, particularly its neuroprotective, antioxidant, and stress-protective properties. This article synthesizes findings from various studies to provide a comprehensive overview of the biological activity of this compound.
Chemical Structure and Properties
The empirical formula of magnesium comenate is established as . The compound crystallizes with eight water molecules, six of which are part of the hydration shell surrounding the magnesium cation. The structure is stabilized by a network of hydrogen bonds involving both solvate water molecules and functional groups of ionized ligand molecules .
In Vitro Studies
In vitro studies have demonstrated that magnesium comenate exhibits significant neuroprotective effects against excitotoxicity induced by glutamate. At concentrations ranging from 0.0001 mM to 1 mM, this compound effectively preserved neurite growth parameters under oxidative stress caused by hydrogen peroxide. Specifically, it increased neuron survival rates significantly compared to control groups treated with glutamate, achieving up to 64% survival at a concentration of 0.1 mM .
Concentration (mM) | Neuron Survival (%) |
---|---|
0.001 | 56 |
0.01 | 60 |
0.1 | 64 |
1 | 60 |
In Vivo Studies
In vivo experiments involving animal models have further corroborated these findings. Administration of magnesium comenate at a dose of 2 mg/kg prior to and during stress exposure resulted in a notable decrease in oxidative damage in brain tissues. The compound effectively normalized the antioxidant system during periods of immobilization-cold stress, highlighting its potential therapeutic applications in neuroprotection .
Antioxidant Activity
The antioxidant activity of magnesium comenate was assessed using the "Citrate-Phosphate-Luminol" (CPL) model system. Results indicated a significant reduction in free radical content when compared to control samples, with an increase in concentration leading to enhanced radical quenching efficiency—up to approximately 36% at higher concentrations .
Stress Protective Action
Magnesium comenate also demonstrated stress-protective properties in vivo. Under conditions of induced oxidative stress, the compound significantly mitigated increases in malondialdehyde (MDA) levels—a marker of oxidative damage—suggesting its role in protecting against oxidative processes during stress .
Case Studies
Several case studies have illustrated the practical implications of magnesium comenate's biological activities:
- Case Study 1 : In a controlled study on rats subjected to immobilization stress, administration of magnesium comenate led to a statistically significant reduction in biomarkers associated with oxidative stress compared to untreated control groups.
- Case Study 2 : A comparative analysis of neuron cultures exposed to glutamate showed that those treated with magnesium comenate maintained higher viability and neurite growth compared to cultures treated only with glutamate.
Propriétés
IUPAC Name |
1-[2-hydroxy-3-(4-phenylpiperazin-1-yl)propyl]pyrrolidin-2-one | |
---|---|---|
Source | PubChem | |
URL | https://pubchem.ncbi.nlm.nih.gov | |
Description | Data deposited in or computed by PubChem | |
InChI |
InChI=1S/C17H25N3O2/c21-16(14-20-8-4-7-17(20)22)13-18-9-11-19(12-10-18)15-5-2-1-3-6-15/h1-3,5-6,16,21H,4,7-14H2 | |
Source | PubChem | |
URL | https://pubchem.ncbi.nlm.nih.gov | |
Description | Data deposited in or computed by PubChem | |
InChI Key |
AFBKGQPVUQUMRC-UHFFFAOYSA-N | |
Source | PubChem | |
URL | https://pubchem.ncbi.nlm.nih.gov | |
Description | Data deposited in or computed by PubChem | |
Canonical SMILES |
C1CC(=O)N(C1)CC(CN2CCN(CC2)C3=CC=CC=C3)O | |
Source | PubChem | |
URL | https://pubchem.ncbi.nlm.nih.gov | |
Description | Data deposited in or computed by PubChem | |
Molecular Formula |
C17H25N3O2 | |
Source | PubChem | |
URL | https://pubchem.ncbi.nlm.nih.gov | |
Description | Data deposited in or computed by PubChem | |
DSSTOX Substance ID |
DTXSID20933361 | |
Record name | 1-[2-Hydroxy-3-(4-phenylpiperazin-1-yl)propyl]pyrrolidin-2-one | |
Source | EPA DSSTox | |
URL | https://comptox.epa.gov/dashboard/DTXSID20933361 | |
Description | DSSTox provides a high quality public chemistry resource for supporting improved predictive toxicology. | |
Molecular Weight |
303.4 g/mol | |
Source | PubChem | |
URL | https://pubchem.ncbi.nlm.nih.gov | |
Description | Data deposited in or computed by PubChem | |
CAS No. |
148274-76-4 | |
Record name | N-(beta-Hydroxy-gamma-(N-phenylpiperazinepropyl))-2-pyrrolidinone | |
Source | ChemIDplus | |
URL | https://pubchem.ncbi.nlm.nih.gov/substance/?source=chemidplus&sourceid=0148274764 | |
Description | ChemIDplus is a free, web search system that provides access to the structure and nomenclature authority files used for the identification of chemical substances cited in National Library of Medicine (NLM) databases, including the TOXNET system. | |
Record name | 1-[2-Hydroxy-3-(4-phenylpiperazin-1-yl)propyl]pyrrolidin-2-one | |
Source | EPA DSSTox | |
URL | https://comptox.epa.gov/dashboard/DTXSID20933361 | |
Description | DSSTox provides a high quality public chemistry resource for supporting improved predictive toxicology. | |
Avertissement et informations sur les produits de recherche in vitro
Veuillez noter que tous les articles et informations sur les produits présentés sur BenchChem sont destinés uniquement à des fins informatives. Les produits disponibles à l'achat sur BenchChem sont spécifiquement conçus pour des études in vitro, qui sont réalisées en dehors des organismes vivants. Les études in vitro, dérivées du terme latin "in verre", impliquent des expériences réalisées dans des environnements de laboratoire contrôlés à l'aide de cellules ou de tissus. Il est important de noter que ces produits ne sont pas classés comme médicaments et n'ont pas reçu l'approbation de la FDA pour la prévention, le traitement ou la guérison de toute condition médicale, affection ou maladie. Nous devons souligner que toute forme d'introduction corporelle de ces produits chez les humains ou les animaux est strictement interdite par la loi. Il est essentiel de respecter ces directives pour assurer la conformité aux normes légales et éthiques en matière de recherche et d'expérimentation.