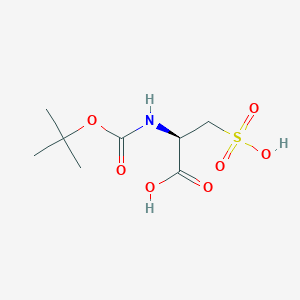
Boc-L-cysteic acid
Vue d'ensemble
Mécanisme D'action
Target of Action
Boc-L-cysteic acid is primarily used in peptide and protein science as a protecting group for the cysteine thiol group . It enables a vast array of peptide and protein chemistry, including the synthesis of complex disulfide-rich peptides, semisynthesis of proteins, and peptide/protein labeling in vitro and in vivo .
Mode of Action
The this compound acts as a protecting group for the cysteine thiol group, facilitating increasingly sophisticated strategies for the protection, and subsequent deprotection, of cysteine . This allows for the synthesis of complex disulfide-rich peptides and proteins .
Biochemical Pathways
The biosynthesis of L-cysteine from L-serine in enteric bacteria such as E. coli and Salmonella typhimurium proceeds via a two-step pathway . L-Serine undergoes a substitution of its β-hydroxyl with a thiol in two steps . This compound, as a protecting group, plays a crucial role in these biochemical pathways.
Pharmacokinetics
It is known that the compound is soluble in water , which suggests it could have good bioavailability. More research is needed to fully understand the ADME (Absorption, Distribution, Metabolism, and Excretion) properties of this compound.
Result of Action
The primary result of this compound’s action is the facilitation of peptide and protein synthesis. By acting as a protecting group for the cysteine thiol group, it enables the synthesis of complex disulfide-rich peptides and proteins . This has significant implications for biochemical research and drug development.
Action Environment
The action of this compound is influenced by various environmental factors. For instance, the compound’s solubility in water suggests that it may be more effective in aqueous environments. Additionally, the compound’s stability may be affected by factors such as temperature and pH, although more research is needed to fully understand these influences.
Analyse Biochimique
Biochemical Properties
Boc-L-cysteic acid plays a significant role in biochemical reactions due to its structural similarity to cysteine. It interacts with various enzymes, proteins, and other biomolecules. One of the primary interactions is with cysteine sulfinic acid decarboxylase, an enzyme that catalyzes the conversion of cysteine sulfinic acid to hypotaurine. This compound can also interact with proteins that contain disulfide bonds, influencing their folding and stability .
Cellular Effects
This compound affects various types of cells and cellular processes. It influences cell function by modulating cell signaling pathways, gene expression, and cellular metabolism. For instance, this compound can alter the redox state of cells by interacting with thiol-containing proteins, thereby affecting cellular signaling pathways that rely on redox regulation. Additionally, it can impact gene expression by modifying the activity of transcription factors that are sensitive to changes in the cellular redox state .
Molecular Mechanism
The molecular mechanism of this compound involves its interaction with biomolecules at the molecular level. It can bind to enzymes and inhibit or activate their activity. For example, this compound can inhibit the activity of cysteine proteases by forming a covalent bond with the active site cysteine residue. This inhibition can lead to changes in protein degradation and turnover. Additionally, this compound can influence gene expression by modifying the activity of transcription factors through redox-dependent mechanisms .
Temporal Effects in Laboratory Settings
In laboratory settings, the effects of this compound can change over time. The compound is relatively stable at room temperature, but it can degrade under certain conditions, such as exposure to strong acids or bases. Long-term effects on cellular function have been observed in both in vitro and in vivo studies. For example, prolonged exposure to this compound can lead to changes in cellular metabolism and gene expression, which may result in altered cell growth and differentiation .
Dosage Effects in Animal Models
The effects of this compound vary with different dosages in animal models. At low doses, it can enhance cellular function by modulating redox-sensitive signaling pathways. At high doses, this compound can exhibit toxic effects, such as oxidative stress and cell death. Threshold effects have been observed, where a specific dosage range is required to achieve the desired biochemical effects without causing toxicity .
Metabolic Pathways
This compound is involved in several metabolic pathways. It can be metabolized to taurine through the action of cysteine sulfinic acid decarboxylase. This conversion is crucial for maintaining cellular redox balance and supporting various physiological functions. Additionally, this compound can influence metabolic flux by modulating the activity of enzymes involved in sulfur amino acid metabolism .
Transport and Distribution
Within cells and tissues, this compound is transported and distributed through specific transporters and binding proteins. It can interact with amino acid transporters, such as the cystine/glutamate antiporter, to facilitate its uptake into cells. Once inside the cell, this compound can bind to proteins and other biomolecules, affecting its localization and accumulation .
Subcellular Localization
This compound is localized to specific subcellular compartments, where it exerts its biochemical effects. It can be directed to the cytoplasm, mitochondria, or other organelles through targeting signals or post-translational modifications. The subcellular localization of this compound can influence its activity and function, as it interacts with different biomolecules in distinct cellular environments .
Méthodes De Préparation
Synthetic Routes and Reaction Conditions
Boc-L-cysteic acid is typically synthesized through the protection of the amino group of L-cysteic acid using tert-butoxycarbonyl (Boc) groups. The process involves the reaction of L-cysteic acid with di-tert-butyl dicarbonate (Boc2O) in the presence of a base such as triethylamine. The reaction is carried out under anhydrous conditions to prevent hydrolysis .
Industrial Production Methods
Industrial production of this compound follows similar synthetic routes but on a larger scale. The process involves the use of automated peptide synthesizers and large-scale reactors to ensure high yield and purity. The reaction conditions are optimized to minimize side reactions and maximize the efficiency of the Boc protection .
Analyse Des Réactions Chimiques
Types of Reactions
Boc-L-cysteic acid undergoes various chemical reactions, including:
Oxidation: The sulfo group can be oxidized to form sulfonic acids.
Reduction: The sulfo group can be reduced to form thiols.
Substitution: The Boc group can be substituted with other protecting groups under acidic conditions
Common Reagents and Conditions
Oxidation: Hydrogen peroxide or potassium permanganate.
Reduction: Dithiothreitol (DTT) or tris(2-carboxyethyl)phosphine (TCEP).
Substitution: Trifluoroacetic acid (TFA) for Boc deprotection.
Major Products Formed
Applications De Recherche Scientifique
Boc-L-cysteic acid has a wide range of applications in scientific research:
Chemistry: Used in the synthesis of peptides and proteins, particularly in the protection of amino groups during peptide synthesis.
Biology: Utilized in the study of protein folding and structure due to its ability to form disulfide bonds.
Medicine: Investigated for its potential in drug development and as a building block for therapeutic peptides.
Industry: Employed in the production of specialty chemicals and as a reagent in various chemical processes.
Comparaison Avec Des Composés Similaires
Similar Compounds
Fmoc-L-cysteic acid: Another protected form of L-cysteic acid, using fluorenylmethyloxycarbonyl (Fmoc) as the protecting group.
DNP-L-cysteic acid: Uses dinitrophenyl (DNP) as the protecting group.
N-acetyl-L-cysteic acid: Uses an acetyl group for protection
Uniqueness
Boc-L-cysteic acid is unique due to its Boc protecting group, which offers stability under basic conditions and selective deprotection under acidic conditions. This makes it particularly useful in solid-phase peptide synthesis and other applications where selective protection and deprotection are crucial .
Propriétés
IUPAC Name |
(2R)-2-[(2-methylpropan-2-yl)oxycarbonylamino]-3-sulfopropanoic acid | |
---|---|---|
Source | PubChem | |
URL | https://pubchem.ncbi.nlm.nih.gov | |
Description | Data deposited in or computed by PubChem | |
InChI |
InChI=1S/C8H15NO7S/c1-8(2,3)16-7(12)9-5(6(10)11)4-17(13,14)15/h5H,4H2,1-3H3,(H,9,12)(H,10,11)(H,13,14,15)/t5-/m0/s1 | |
Source | PubChem | |
URL | https://pubchem.ncbi.nlm.nih.gov | |
Description | Data deposited in or computed by PubChem | |
InChI Key |
OBDTYOCDTWYTGV-YFKPBYRVSA-N | |
Source | PubChem | |
URL | https://pubchem.ncbi.nlm.nih.gov | |
Description | Data deposited in or computed by PubChem | |
Canonical SMILES |
CC(C)(C)OC(=O)NC(CS(=O)(=O)O)C(=O)O | |
Source | PubChem | |
URL | https://pubchem.ncbi.nlm.nih.gov | |
Description | Data deposited in or computed by PubChem | |
Isomeric SMILES |
CC(C)(C)OC(=O)N[C@@H](CS(=O)(=O)O)C(=O)O | |
Source | PubChem | |
URL | https://pubchem.ncbi.nlm.nih.gov | |
Description | Data deposited in or computed by PubChem | |
Molecular Formula |
C8H15NO7S | |
Source | PubChem | |
URL | https://pubchem.ncbi.nlm.nih.gov | |
Description | Data deposited in or computed by PubChem | |
Molecular Weight |
269.27 g/mol | |
Source | PubChem | |
URL | https://pubchem.ncbi.nlm.nih.gov | |
Description | Data deposited in or computed by PubChem | |
Retrosynthesis Analysis
AI-Powered Synthesis Planning: Our tool employs the Template_relevance Pistachio, Template_relevance Bkms_metabolic, Template_relevance Pistachio_ringbreaker, Template_relevance Reaxys, Template_relevance Reaxys_biocatalysis model, leveraging a vast database of chemical reactions to predict feasible synthetic routes.
One-Step Synthesis Focus: Specifically designed for one-step synthesis, it provides concise and direct routes for your target compounds, streamlining the synthesis process.
Accurate Predictions: Utilizing the extensive PISTACHIO, BKMS_METABOLIC, PISTACHIO_RINGBREAKER, REAXYS, REAXYS_BIOCATALYSIS database, our tool offers high-accuracy predictions, reflecting the latest in chemical research and data.
Strategy Settings
Precursor scoring | Relevance Heuristic |
---|---|
Min. plausibility | 0.01 |
Model | Template_relevance |
Template Set | Pistachio/Bkms_metabolic/Pistachio_ringbreaker/Reaxys/Reaxys_biocatalysis |
Top-N result to add to graph | 6 |
Feasible Synthetic Routes
Avertissement et informations sur les produits de recherche in vitro
Veuillez noter que tous les articles et informations sur les produits présentés sur BenchChem sont destinés uniquement à des fins informatives. Les produits disponibles à l'achat sur BenchChem sont spécifiquement conçus pour des études in vitro, qui sont réalisées en dehors des organismes vivants. Les études in vitro, dérivées du terme latin "in verre", impliquent des expériences réalisées dans des environnements de laboratoire contrôlés à l'aide de cellules ou de tissus. Il est important de noter que ces produits ne sont pas classés comme médicaments et n'ont pas reçu l'approbation de la FDA pour la prévention, le traitement ou la guérison de toute condition médicale, affection ou maladie. Nous devons souligner que toute forme d'introduction corporelle de ces produits chez les humains ou les animaux est strictement interdite par la loi. Il est essentiel de respecter ces directives pour assurer la conformité aux normes légales et éthiques en matière de recherche et d'expérimentation.