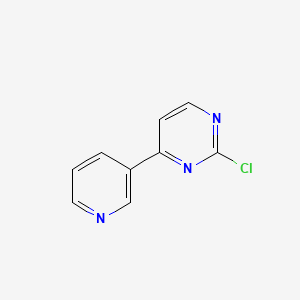
2-Chloro-4-(pyridin-3-yl)pyrimidine
Vue d'ensemble
Description
2-Chloro-4-(pyridin-3-yl)pyrimidine is a chemical compound with the molecular formula C9H6ClN3 . It undergoes cobalt-catalyzed cross-coupling reaction with aryl halides .
Synthesis Analysis
The synthesis of 2-Chloro-4-(pyridin-3-yl)pyrimidine involves various methods. For instance, it has been used in the design and synthesis of pyrrolo[2,3-d]pyrimidine-derived leucine-rich repeat kinase 2 (LRRK2) inhibitors . Additionally, a series of novel N-(pyridin-3-yl)pyrimidin-4-amine derivatives were designed and synthesized as potent CDK2 inhibitors .Molecular Structure Analysis
The molecular structure of 2-Chloro-4-(pyridin-3-yl)pyrimidine consists of a pyrimidine ring attached to a pyridine ring via a carbon atom. The pyrimidine ring has a chlorine atom attached to it .Chemical Reactions Analysis
2-Chloro-4-(pyridin-3-yl)pyrimidine undergoes various chemical reactions. For instance, it undergoes a challenging coupling of 2-pyridyl nucleophiles with (hetero)aryl electrophiles . It also reacts under certain conditions with dichloro (1,1’-bis (diphenylphosphanyl)ferrocene)palladium (II)*CH 2 Cl 2 and caesium carbonate in tetrahydrofuran .Physical And Chemical Properties Analysis
2-Chloro-4-(pyridin-3-yl)pyrimidine has a molecular weight of 191.62 g/mol. It has a computed XLogP3-AA value of 1.8, indicating its lipophilicity. It has no hydrogen bond donors, three hydrogen bond acceptors, and one rotatable bond . Its exact mass and monoisotopic mass are 191.0250249 g/mol .Applications De Recherche Scientifique
Anti-Inflammatory Applications
Pyrimidines, including 2-Chloro-4-(pyridin-3-yl)pyrimidine, have been found to exhibit a range of pharmacological effects including anti-inflammatory activities . They are known to inhibit the expression and activities of certain vital inflammatory mediators such as prostaglandin E2, inducible nitric oxide synthase, tumor necrosis factor-α, nuclear factor κB, leukotrienes, and some interleukins .
Synthesis of Novel Compounds
2-Chloro-4-(pyridin-3-yl)pyrimidine has been used in the synthesis of novel bis(2-(pyrimidin-2-yl)ethoxy)alkanes . These novel compounds could potentially have a wide range of applications in various fields of research.
Biosensor for Protein Assay
This compound has been used in the synthesis of a 4′-(1,1′-(5-(2-methoxyphenoxy)-[2,2′-bipyrimidine]-4,6-diyl)bis(1H-pyrazol-3,1-diyl))dianiline fluorescent dye, which is a biosensor for protein assay . This application is particularly important in biological and medical research.
NF-κB and AP-1 Inhibitors
Through solution-phase parallel synthesis and high throughput evaluation, several new 2-chloro-4-(trifluoromethyl)pyrimidine-5-N-(3′,5′-bis(trifluoromethyl)phenyl) carboxamides were recognized as NF-κB and AP-1 inhibitors . These inhibitors play a crucial role in regulating the immune response to infection.
Anti-Diabetic Applications
Due to the efficacy of pyrimidine derivatives to reduce blood glucose, they may find application in the prevention and treatment of disorders involving elevated plasma blood glucose, such as hyperglycemia and ailments in which such a reduction of blood glucose is beneficial .
Cardiovascular Disease Treatment
The same blood glucose-lowering effect of pyrimidine derivatives can also be beneficial in the treatment of cardiovascular diseases . This is because high blood glucose levels can lead to damage to the blood vessels and the heart.
Mécanisme D'action
Target of Action
Pyrimidine derivatives are known to interact with a variety of biological targets, including enzymes and receptors, contributing to their diverse pharmacological effects .
Mode of Action
Pyrimidine derivatives are known to interact with their targets in a variety of ways, often involving the formation of hydrogen bonds and hydrophobic interactions .
Biochemical Pathways
Pyrimidine derivatives are known to influence a variety of biochemical pathways, often related to their target’s function .
Pharmacokinetics
It is known that the compound has high gastrointestinal absorption and is predicted to be bbb permeant . The compound’s lipophilicity (Log Po/w) is reported to be 1.85 (iLOGP) and 1.76 (XLOGP3), which may influence its distribution and bioavailability .
Result of Action
Pyrimidine derivatives are known to have a range of effects at the molecular and cellular level, often related to their target’s function .
Action Environment
It is known that the compound should be stored under an inert gas (nitrogen or argon) at 2-8°c .
Orientations Futures
Propriétés
IUPAC Name |
2-chloro-4-pyridin-3-ylpyrimidine | |
---|---|---|
Source | PubChem | |
URL | https://pubchem.ncbi.nlm.nih.gov | |
Description | Data deposited in or computed by PubChem | |
InChI |
InChI=1S/C9H6ClN3/c10-9-12-5-3-8(13-9)7-2-1-4-11-6-7/h1-6H | |
Source | PubChem | |
URL | https://pubchem.ncbi.nlm.nih.gov | |
Description | Data deposited in or computed by PubChem | |
InChI Key |
MGQROXOMFRGAOY-UHFFFAOYSA-N | |
Source | PubChem | |
URL | https://pubchem.ncbi.nlm.nih.gov | |
Description | Data deposited in or computed by PubChem | |
Canonical SMILES |
C1=CC(=CN=C1)C2=NC(=NC=C2)Cl | |
Source | PubChem | |
URL | https://pubchem.ncbi.nlm.nih.gov | |
Description | Data deposited in or computed by PubChem | |
Molecular Formula |
C9H6ClN3 | |
Source | PubChem | |
URL | https://pubchem.ncbi.nlm.nih.gov | |
Description | Data deposited in or computed by PubChem | |
DSSTOX Substance ID |
DTXSID20473090 | |
Record name | 2-Chloro-4-(pyridin-3-yl)pyrimidine | |
Source | EPA DSSTox | |
URL | https://comptox.epa.gov/dashboard/DTXSID20473090 | |
Description | DSSTox provides a high quality public chemistry resource for supporting improved predictive toxicology. | |
Molecular Weight |
191.62 g/mol | |
Source | PubChem | |
URL | https://pubchem.ncbi.nlm.nih.gov | |
Description | Data deposited in or computed by PubChem | |
Product Name |
2-Chloro-4-(pyridin-3-yl)pyrimidine | |
CAS RN |
483324-01-2 | |
Record name | 2-Chloro-4-(pyridin-3-yl)pyrimidine | |
Source | EPA DSSTox | |
URL | https://comptox.epa.gov/dashboard/DTXSID20473090 | |
Description | DSSTox provides a high quality public chemistry resource for supporting improved predictive toxicology. | |
Record name | 2-chloro-4-(pyridin-3-yl)pyrimidine | |
Source | European Chemicals Agency (ECHA) | |
URL | https://echa.europa.eu/information-on-chemicals | |
Description | The European Chemicals Agency (ECHA) is an agency of the European Union which is the driving force among regulatory authorities in implementing the EU's groundbreaking chemicals legislation for the benefit of human health and the environment as well as for innovation and competitiveness. | |
Explanation | Use of the information, documents and data from the ECHA website is subject to the terms and conditions of this Legal Notice, and subject to other binding limitations provided for under applicable law, the information, documents and data made available on the ECHA website may be reproduced, distributed and/or used, totally or in part, for non-commercial purposes provided that ECHA is acknowledged as the source: "Source: European Chemicals Agency, http://echa.europa.eu/". Such acknowledgement must be included in each copy of the material. ECHA permits and encourages organisations and individuals to create links to the ECHA website under the following cumulative conditions: Links can only be made to webpages that provide a link to the Legal Notice page. | |
Synthesis routes and methods I
Procedure details
Synthesis routes and methods II
Procedure details
Synthesis routes and methods III
Procedure details
Retrosynthesis Analysis
AI-Powered Synthesis Planning: Our tool employs the Template_relevance Pistachio, Template_relevance Bkms_metabolic, Template_relevance Pistachio_ringbreaker, Template_relevance Reaxys, Template_relevance Reaxys_biocatalysis model, leveraging a vast database of chemical reactions to predict feasible synthetic routes.
One-Step Synthesis Focus: Specifically designed for one-step synthesis, it provides concise and direct routes for your target compounds, streamlining the synthesis process.
Accurate Predictions: Utilizing the extensive PISTACHIO, BKMS_METABOLIC, PISTACHIO_RINGBREAKER, REAXYS, REAXYS_BIOCATALYSIS database, our tool offers high-accuracy predictions, reflecting the latest in chemical research and data.
Strategy Settings
Precursor scoring | Relevance Heuristic |
---|---|
Min. plausibility | 0.01 |
Model | Template_relevance |
Template Set | Pistachio/Bkms_metabolic/Pistachio_ringbreaker/Reaxys/Reaxys_biocatalysis |
Top-N result to add to graph | 6 |
Feasible Synthetic Routes
Avertissement et informations sur les produits de recherche in vitro
Veuillez noter que tous les articles et informations sur les produits présentés sur BenchChem sont destinés uniquement à des fins informatives. Les produits disponibles à l'achat sur BenchChem sont spécifiquement conçus pour des études in vitro, qui sont réalisées en dehors des organismes vivants. Les études in vitro, dérivées du terme latin "in verre", impliquent des expériences réalisées dans des environnements de laboratoire contrôlés à l'aide de cellules ou de tissus. Il est important de noter que ces produits ne sont pas classés comme médicaments et n'ont pas reçu l'approbation de la FDA pour la prévention, le traitement ou la guérison de toute condition médicale, affection ou maladie. Nous devons souligner que toute forme d'introduction corporelle de ces produits chez les humains ou les animaux est strictement interdite par la loi. Il est essentiel de respecter ces directives pour assurer la conformité aux normes légales et éthiques en matière de recherche et d'expérimentation.