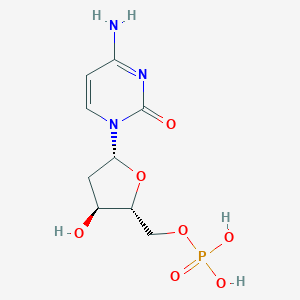
2'-Deoxycytidine-5'-monophosphoric acid
Vue d'ensemble
Description
2'-Deoxycytidine-5'-monophosphoric acid (dCMP) is a deoxyribonucleotide integral to DNA biosynthesis. Structurally, it consists of a cytosine base, a deoxyribose sugar, and a monophosphate group esterified at the 5'-position (CAS: 1032-65-1; MW: 307.2 g/mol) . dCMP serves as a substrate for uridine/cytidine monophosphate kinases (UMP/CMP kinase, EC 2.7.4.4), which phosphorylate it to dCDP and subsequently to dCTP for incorporation into DNA . Its solubility in water and stability under recommended storage conditions (-20°C in inert atmospheres) make it a critical reagent in molecular biology and enzymology studies .
Méthodes De Préparation
Synthetic Routes and Reaction Conditions
2’-Deoxycytidine-5’-monophosphate can be synthesized through enzymatic phosphorylation of 2’-deoxycytidine using deoxycytidine kinase or deoxynucleoside kinase . These enzymes facilitate the transfer of a phosphate group to the 5’ position of the deoxynucleoside, resulting in the formation of the monophosphate.
Industrial Production Methods
Industrial production of 2’-Deoxycytidine-5’-monophosphate typically involves the extraction of DNA from biological sources, followed by enzymatic hydrolysis to obtain the desired nucleotide . This method ensures high yield and purity, making it suitable for large-scale production.
Analyse Des Réactions Chimiques
Enzymatic Phosphorylation to dCDP and dCTP
dCMP serves as a substrate for kinases in nucleotide metabolism. Key reactions include:
-
Phosphorylation by UMP/CMP kinase (EC 2.7.4.4):
dCMP is converted to dCDP (deoxycytidine diphosphate), which is further phosphorylated to dCTP (deoxycytidine triphosphate) for DNA synthesis . -
Kinase Activity:
Enzymes like Drosophila melanogaster deoxynucleoside kinase (dNK) and Bacillus subtilis deoxycytidine kinase (dCK) phosphorylate dCMP using guanosine triphosphate (GTP) as a phosphate donor .
Table 1: Enzymatic Phosphorylation Efficiency
Enzyme | Substrate | Phosphate Donor | Specific Activity (µmol·min⁻¹·mg⁻¹) | Yield (%) | Source |
---|---|---|---|---|---|
dNK | 2'-Deoxycytidine | GTP | 35.9 | 95 | |
dCK | 2'-Deoxycytidine | GTP | 1.6 | 90 |
Inhibition of Thymidylate Synthase (TS)
dCMP derivatives, such as 5-fluoro-2'-deoxycytidine 5'-monophosphate (FdCMP), act as mechanism-based inhibitors of TS, an enzyme critical for dTMP synthesis :
-
Mechanism: FdCMP forms a ternary complex with TS and 5,10-methylene-tetrahydrofolate (CH₂H₄folate), leading to irreversible enzyme inhibition .
-
Kinetic Parameters:
Role in Mutagenesis via Modified Derivatives
Modified dCMP analogs, such as 5-chloro-2'-deoxycytidine (5CldC), exhibit mutagenic properties when incorporated into DNA:
-
Mutational Spectrum:
-
Biological Impact:
Table 2: Mutational Profile of 5CldC in MEFs
Mutation Type | Frequency (%) | Context Bias | Source |
---|---|---|---|
CG → TA | 86.2 | 5'-GC(Y)-3' | |
TA → CG | 7.9 | Non-CpG sites |
Interaction with DNA Polymerases
dCMP derivatives serve as substrates for DNA polymerases:
-
HIV-1 Reverse Transcriptase (RT):
Chemical Stability and Degradation
Applications De Recherche Scientifique
Molecular Biology and Nucleotide Synthesis
Role in DNA and RNA Biosynthesis
- dCMP is an essential substrate for the synthesis of deoxyribonucleic acid (DNA). It is phosphorylated to form dCDP (deoxycytidine diphosphate) and subsequently to dCTP (deoxycytidine triphosphate), which is incorporated into DNA strands during replication and repair processes .
- The enzyme uridine monophosphate/cytidine monophosphate kinase catalyzes the conversion of dCMP to dCDP, highlighting its importance in nucleotide metabolism .
Biochemical Assays
- dCMP is utilized in various biochemical assays to study enzyme kinetics and activity. For example, a continuous spectrophotometric assay has been developed to measure deoxycytidine kinase activity by coupling the formation of nucleoside 5'-monophosphate to a methylation reaction, allowing for real-time monitoring of enzymatic activity .
Cancer Research and Treatment
Epigenetic Therapy
- 5-Aza-2'-deoxycytidine (decitabine), a derivative of dCMP, is an FDA-approved drug used in the treatment of myelodysplastic syndromes and acute myeloid leukemia. It acts as a DNA methyltransferase inhibitor, reversing aberrant DNA methylation patterns that silence tumor suppressor genes .
- Research indicates that decitabine can be delivered effectively using oligonucleotides containing dCMP derivatives, enhancing its therapeutic efficacy while minimizing degradation by cellular enzymes .
Mechanism of Action
- The incorporation of modified nucleotides like 5-fluoro-2'-deoxycytidine into DNA has been shown to inhibit DNA methylation and induce expression of tumor suppressor genes, further establishing the significance of dCMP-related compounds in cancer therapy .
Drug Development and Therapeutics
Nucleotide Analogues
- Novel nucleotide analogues based on dCMP are being developed for antiviral therapies targeting viral polymerases. These compounds undergo phosphorylation to become active triphosphates that can be incorporated into viral DNA, effectively inhibiting viral replication .
- Research has identified specific structural modifications that enhance the efficacy and selectivity of these analogues against various viral targets, showcasing the versatility of dCMP derivatives in drug design .
Enzymatic Studies
Thymidylate Synthase Activity
- dCMP serves as an important substrate for thymidylate synthase, which catalyzes the conversion of deoxyuridine monophosphate (dUMP) to deoxythymidine monophosphate (dTMP). This reaction is vital for DNA synthesis and repair, making dCMP critical in studying enzyme kinetics related to nucleotide metabolism .
Kinetic Studies
Mécanisme D'action
2’-Deoxycytidine-5’-monophosphate exerts its effects primarily through its role as a substrate for kinases involved in DNA and RNA synthesis . It is phosphorylated to form 2’-deoxycytidine-5’-diphosphate and 2’-deoxycytidine-5’-triphosphate, which are then incorporated into DNA and RNA strands . Additionally, it can undergo deamination to form 2’-deoxyuridine-5’-monophosphate, which is involved in DNA repair pathways .
Comparaison Avec Des Composés Similaires
Structural and Functional Comparisons
Table 1: Key Properties of dCMP and Analogous Nucleotides
Key Differences :
- Sugar Backbone : dCMP, dUMP, and dTMP contain deoxyribose, while CMP has ribose, limiting CMP to RNA synthesis .
- Base Specificity : Cytosine in dCMP vs. uracil in dUMP and thymine in dTMP. Thymine’s methyl group (absent in uracil) enhances DNA stability .
- Phosphorylation Pathways : dCMP is phosphorylated to dCTP for DNA, whereas dUMP is converted to dTMP via thymidylate synthase, a target for chemotherapeutic agents like 5-Fluorouracil .
Biochemical Interactions and Inhibition
- dCTP and dCMP inhibit thymidine kinase (competitive inhibition; Ki = 7.6 × 10<sup>-6</sup> M for dCTP), regulating nucleotide pool balance .
- Modified Analogs :
- 5hmdC : Synthesized via P(V)-N activation or enzymatic conversion from dCMP, 5hmdC is critical in epigenetic regulation. Its triphosphate form (5hmdCTP) is incorporated into DNA during replication .
- C5-Modified dCMP Derivatives : Polyphosphorylated analogs (e.g., C5-azido-dCMP) exhibit enhanced polymerase compatibility and are used in click chemistry-based DNA labeling .
Activité Biologique
2'-Deoxycytidine-5'-monophosphoric acid (dCMP) is a critical nucleotide involved in DNA synthesis and repair. It serves as a building block for DNA, playing a significant role in various biological processes, including cellular proliferation, immune responses, and epigenetic regulation. This article reviews the biological activities of dCMP, emphasizing its enzymatic interactions, therapeutic applications, and implications in disease contexts.
2'-Deoxycytidine-5'-monophosphate is a pyrimidine nucleoside monophosphate composed of a deoxyribose sugar linked to a cytosine base, with a phosphate group attached to the 5' carbon. Its structure is essential for its function in DNA synthesis and metabolism.
Property | Value |
---|---|
Molecular Formula | C9H13N3O4P |
Molecular Weight | 243.18 g/mol |
Solubility | Soluble in water |
Stability | Sensitive to hydrolysis |
1. Role in DNA Synthesis
dCMP is phosphorylated to form dCDP and subsequently dCTP, which are essential substrates for DNA polymerases during DNA replication. The enzyme UMP-CMP kinase catalyzes the conversion of dCMP to dCDP, highlighting its role in nucleotide metabolism .
2. Enzymatic Interactions
dCMP interacts with various enzymes that regulate its metabolism:
- Deoxycytidine Kinase : Converts deoxycytidine to dCMP, crucial for activating antiviral and anticancer agents .
- dCMP Deaminase : Catalyzes the deamination of dCMP to uridine monophosphate (UMP), influencing nucleotide pool balance .
3. Immunological Activity
Recent studies indicate that dCMP can enhance immune cell proliferation in response to influenza virus antigens, suggesting its potential role as an immunomodulator . This activity may be leveraged in therapeutic settings to boost immune responses against infections or tumors.
1. Anticancer Therapy
dCMP and its analogs, such as 5-Aza-2'-deoxycytidine (decitabine), are employed in epigenetic therapy to reverse aberrant DNA methylation in cancer cells. These compounds inhibit DNA methyltransferases (DNMTs), restoring the expression of tumor suppressor genes .
Case Study: Decitabine Treatment
In clinical trials, decitabine has shown efficacy in treating myelodysplastic syndromes by reactivating silenced genes through demethylation processes .
2. Antiviral Agents
The metabolism of dCMP is crucial for developing antiviral drugs. Increased activity of deoxycytidine kinase correlates with enhanced efficacy of nucleoside analogs used in antiviral therapies .
Enzymatic Kinetics
Studies have demonstrated the kinetic properties of dCMP when interacting with various enzymes:
Q & A
Basic Research Questions
Q. What is the enzymatic role of dCMP in DNA biosynthesis, and how can this be experimentally validated?
dCMP serves as a substrate for uridine/cytidine monophosphate kinases (UMP/CMP kinase, EC 2.7.4.4), which phosphorylate it to dCDP. Subsequent phosphorylation by nucleoside diphosphate kinases generates dCTP, a direct precursor for DNA synthesis. To validate this pathway:
- Perform in vitro kinase assays using purified enzymes, ATP as a phosphate donor, and HPLC or radiometric methods to quantify dCDP/dCTP formation .
- Use CRISPR-Cas9 knockout cell lines to silence kinase expression and measure dCTP pool depletion via LC-MS .
Q. What safety protocols are critical when handling dCMP in laboratory settings?
- PPE Requirements : Wear nitrile gloves, safety goggles, and P95 respirators to avoid inhalation of aerosols (OSHA/NIOSH standards) .
- Storage : Store at -20°C in airtight containers under inert gas (e.g., argon) to prevent degradation; note discrepancies in recommended temperatures (-10°C vs. -20°C across studies) .
- Spill Management : Collect spills using vacuum systems with HEPA filters to minimize dust dispersion; avoid aqueous washdowns to prevent drainage contamination .
Q. How do dCMP’s physical properties influence experimental design?
- Solubility : dCMP is water-soluble (≥200 mg/mL), making it suitable for aqueous reaction buffers. For organic-phase studies (e.g., lipid nanoparticle encapsulation), use DMSO with caution due to partial solubility .
- Stability : Decomposes at 169–172°C; avoid heating above 60°C in enzymatic assays. UV-Vis monitoring at λmax = 277 nm ensures integrity during long-term experiments .
Advanced Research Questions
Q. How can researchers resolve contradictions in reported enzymatic activity of dCMP across species?
Discrepancies in kinase affinity (e.g., human vs. bacterial enzymes) may arise from:
- Cofactor Requirements : Test Mg²⁺/Mn²⁺ concentrations (1–10 mM) to optimize phosphorylation rates .
- Structural Variants : Use X-ray crystallography (resolution ≤2.0 Å) to compare active-site conformations in dCMP-bound kinases .
- Data Normalization : Express activity as "nmol product/min/mg protein" to standardize comparisons .
Q. What synthetic strategies improve yield and purity of dCMP derivatives for structural studies?
- One-Pot Synthesis : A three-step process (phosphorylation, deprotection, purification) achieves 65–70% yield with ≥95% HPLC purity. Key steps:
- Use tert-butyldimethylsilyl (TBDMS) groups to protect the 5'-OH group .
- Purify via ion-exchange chromatography (DEAE Sephadex) to remove unreacted cytidine .
- Isotope Labeling : Incorporate ¹³C/¹⁵N labels via enzymatic transamination for NMR-based interaction studies .
Q. How does dCMP’s metabolic stability impact its utility in long-term cell culture assays?
- Degradation Pathways : dCMP is hydrolyzed by phosphatases to deoxycytidine (t₁/₂ = 4–6 hrs in serum-containing media). Inhibitors like β-glycerophosphate (10 mM) extend stability .
- Cell Uptake : Measure intracellular dCMP levels using LC-MS/MS with deuterated internal standards (e.g., dCMP-d4) .
Q. Contradictions and Limitations
Propriétés
IUPAC Name |
[5-(4-amino-2-oxopyrimidin-1-yl)-3-hydroxyoxolan-2-yl]methyl dihydrogen phosphate | |
---|---|---|
Details | Computed by Lexichem TK 2.7.0 (PubChem release 2021.10.14) | |
Source | PubChem | |
URL | https://pubchem.ncbi.nlm.nih.gov | |
Description | Data deposited in or computed by PubChem | |
InChI |
InChI=1S/C9H14N3O7P/c10-7-1-2-12(9(14)11-7)8-3-5(13)6(19-8)4-18-20(15,16)17/h1-2,5-6,8,13H,3-4H2,(H2,10,11,14)(H2,15,16,17) | |
Details | Computed by InChI 1.0.6 (PubChem release 2021.10.14) | |
Source | PubChem | |
URL | https://pubchem.ncbi.nlm.nih.gov | |
Description | Data deposited in or computed by PubChem | |
InChI Key |
NCMVOABPESMRCP-UHFFFAOYSA-N | |
Details | Computed by InChI 1.0.6 (PubChem release 2021.10.14) | |
Source | PubChem | |
URL | https://pubchem.ncbi.nlm.nih.gov | |
Description | Data deposited in or computed by PubChem | |
Canonical SMILES |
C1C(C(OC1N2C=CC(=NC2=O)N)COP(=O)(O)O)O | |
Details | Computed by OEChem 2.3.0 (PubChem release 2021.10.14) | |
Source | PubChem | |
URL | https://pubchem.ncbi.nlm.nih.gov | |
Description | Data deposited in or computed by PubChem | |
Molecular Formula |
C9H14N3O7P | |
Details | Computed by PubChem 2.2 (PubChem release 2021.10.14) | |
Source | PubChem | |
URL | https://pubchem.ncbi.nlm.nih.gov | |
Description | Data deposited in or computed by PubChem | |
DSSTOX Substance ID |
DTXSID70862516 | |
Record name | 4-Amino-1-(2-deoxy-5-O-phosphonopentofuranosyl)pyrimidin-2(1H)-one | |
Source | EPA DSSTox | |
URL | https://comptox.epa.gov/dashboard/DTXSID70862516 | |
Description | DSSTox provides a high quality public chemistry resource for supporting improved predictive toxicology. | |
Molecular Weight |
307.20 g/mol | |
Details | Computed by PubChem 2.2 (PubChem release 2021.10.14) | |
Source | PubChem | |
URL | https://pubchem.ncbi.nlm.nih.gov | |
Description | Data deposited in or computed by PubChem | |
CAS No. |
1032-65-1 | |
Record name | Cytidine 5'-(dihydrogen phosphate) | |
Source | European Chemicals Agency (ECHA) | |
URL | https://echa.europa.eu/substance-information/-/substanceinfo/100.012.591 | |
Description | The European Chemicals Agency (ECHA) is an agency of the European Union which is the driving force among regulatory authorities in implementing the EU's groundbreaking chemicals legislation for the benefit of human health and the environment as well as for innovation and competitiveness. | |
Explanation | Use of the information, documents and data from the ECHA website is subject to the terms and conditions of this Legal Notice, and subject to other binding limitations provided for under applicable law, the information, documents and data made available on the ECHA website may be reproduced, distributed and/or used, totally or in part, for non-commercial purposes provided that ECHA is acknowledged as the source: "Source: European Chemicals Agency, http://echa.europa.eu/". Such acknowledgement must be included in each copy of the material. ECHA permits and encourages organisations and individuals to create links to the ECHA website under the following cumulative conditions: Links can only be made to webpages that provide a link to the Legal Notice page. | |
Retrosynthesis Analysis
AI-Powered Synthesis Planning: Our tool employs the Template_relevance Pistachio, Template_relevance Bkms_metabolic, Template_relevance Pistachio_ringbreaker, Template_relevance Reaxys, Template_relevance Reaxys_biocatalysis model, leveraging a vast database of chemical reactions to predict feasible synthetic routes.
One-Step Synthesis Focus: Specifically designed for one-step synthesis, it provides concise and direct routes for your target compounds, streamlining the synthesis process.
Accurate Predictions: Utilizing the extensive PISTACHIO, BKMS_METABOLIC, PISTACHIO_RINGBREAKER, REAXYS, REAXYS_BIOCATALYSIS database, our tool offers high-accuracy predictions, reflecting the latest in chemical research and data.
Strategy Settings
Precursor scoring | Relevance Heuristic |
---|---|
Min. plausibility | 0.01 |
Model | Template_relevance |
Template Set | Pistachio/Bkms_metabolic/Pistachio_ringbreaker/Reaxys/Reaxys_biocatalysis |
Top-N result to add to graph | 6 |
Feasible Synthetic Routes
Avertissement et informations sur les produits de recherche in vitro
Veuillez noter que tous les articles et informations sur les produits présentés sur BenchChem sont destinés uniquement à des fins informatives. Les produits disponibles à l'achat sur BenchChem sont spécifiquement conçus pour des études in vitro, qui sont réalisées en dehors des organismes vivants. Les études in vitro, dérivées du terme latin "in verre", impliquent des expériences réalisées dans des environnements de laboratoire contrôlés à l'aide de cellules ou de tissus. Il est important de noter que ces produits ne sont pas classés comme médicaments et n'ont pas reçu l'approbation de la FDA pour la prévention, le traitement ou la guérison de toute condition médicale, affection ou maladie. Nous devons souligner que toute forme d'introduction corporelle de ces produits chez les humains ou les animaux est strictement interdite par la loi. Il est essentiel de respecter ces directives pour assurer la conformité aux normes légales et éthiques en matière de recherche et d'expérimentation.