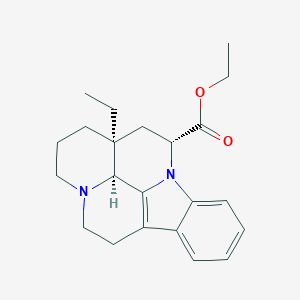
(-)-Éther éthylique de l'acide dihydroapovincaminique
Vue d'ensemble
Description
(-)-(14alpha)-Dihydrovinpocetine: is a synthetic derivative of the alkaloid vincamine, which is extracted from the periwinkle plant (Vinca minor). This compound has garnered significant interest due to its potential therapeutic applications, particularly in the field of neuroprotection and cognitive enhancement.
Applications De Recherche Scientifique
Chemistry:
Catalysis: (-)-(14alpha)-Dihydrovinpocetine is used as a catalyst in certain organic reactions due to its unique structure.
Biology:
Neuroprotection: The compound has shown potential in protecting neurons from oxidative stress and apoptosis.
Cognitive Enhancement: It is being studied for its ability to enhance cognitive functions and memory.
Medicine:
Therapeutic Agent: (-)-(14alpha)-Dihydrovinpocetine is being investigated as a potential therapeutic agent for neurodegenerative diseases such as Alzheimer’s and Parkinson’s disease.
Industry:
Pharmaceuticals: It is used in the formulation of various pharmaceutical products aimed at improving cognitive functions and treating neurodegenerative conditions.
Mécanisme D'action
Target of Action
As an ester, it is likely to interact with enzymes involved in ester hydrolysis .
Mode of Action
Esters, including (-)-Dihydroapovincaminic acid ethyl ester, can undergo hydrolysis in the presence of a dilute acid such as hydrochloric acid or sulfuric acid, acting as the catalyst . The ester reacts with the water present to produce a carboxylic acid and an alcohol . This reaction is reversible, and an equilibrium mixture is produced containing all four substances .
Biochemical Pathways
The hydrolysis of esters, including (-)-Dihydroapovincaminic acid ethyl ester, involves amino acid uptake and transamination, as well as the decarboxylation of α-keto acid resulting in an aldehyde, which is followed by the reduction to higher alcohols .
Pharmacokinetics
It is known that the bioavailability of ethyl esters can be relatively low, primarily due to their high lipophilicity, which influences their ability to reach the unstirred water layers of the gastrointestinal tract for absorption .
Result of Action
Esters in general can disrupt tight junctions, most likely via reactive oxygen species-dependent mechanisms .
Action Environment
The action of (-)-Dihydroapovincaminic acid ethyl ester can be influenced by environmental factors such as temperature and the presence of a dilute acid, which can act as a catalyst for the hydrolysis of the ester .
Méthodes De Préparation
Synthetic Routes and Reaction Conditions: The synthesis of (-)-(14alpha)-Dihydrovinpocetine typically involves multiple steps starting from vincamine. The key steps include:
Reduction of Vincamine: Vincamine is reduced using a suitable reducing agent such as sodium borohydride (NaBH4) to yield (-)-(14alpha)-Dihydrovinpocetine.
Purification: The crude product is then purified using chromatographic techniques to obtain the desired compound in high purity.
Industrial Production Methods: Industrial production of (-)-(14alpha)-Dihydrovinpocetine follows similar synthetic routes but on a larger scale. The process involves:
Bulk Reduction: Large quantities of vincamine are reduced using industrial-scale reactors.
Continuous Purification: Advanced purification techniques such as high-performance liquid chromatography (HPLC) are employed to ensure consistent quality and purity of the final product.
Analyse Des Réactions Chimiques
Types of Reactions:
Oxidation: (-)-(14alpha)-Dihydrovinpocetine can undergo oxidation reactions, typically using oxidizing agents like potassium permanganate (KMnO4).
Reduction: The compound can be further reduced under specific conditions to yield different derivatives.
Substitution: It can participate in substitution reactions where functional groups are replaced by other groups.
Common Reagents and Conditions:
Oxidation: Potassium permanganate (KMnO4) in an acidic medium.
Reduction: Sodium borohydride (NaBH4) in an alcoholic solvent.
Substitution: Various halogenating agents under controlled conditions.
Major Products Formed:
Oxidation: Formation of oxidized derivatives with altered functional groups.
Reduction: Formation of more reduced derivatives.
Substitution: Formation of substituted derivatives with different functional groups.
Comparaison Avec Des Composés Similaires
Vinpocetine: A closely related compound with similar neuroprotective and cognitive-enhancing properties.
Vincamine: The parent compound from which (-)-(14alpha)-Dihydrovinpocetine is derived.
Cinnarizine: Another compound used for its neuroprotective effects.
Uniqueness:
Enhanced Potency: (-)-(14alpha)-Dihydrovinpocetine is considered to have enhanced potency compared to its parent compound vincamine.
Specificity: It has a more specific mechanism of action, particularly in targeting voltage-gated sodium channels.
Activité Biologique
(-)-(14alpha)-Dihydrovinpocetine is a derivative of the alkaloid vincamine, primarily extracted from the periwinkle plant (Vinca minor). This compound has garnered attention for its potential neuroprotective and cognitive-enhancing properties. Its biological activity is attributed to its effects on cerebral blood flow, neurotransmitter modulation, and antioxidant activity. This article explores the biological activity of (-)-(14alpha)-Dihydrovinpocetine through various studies, highlighting its pharmacological effects, mechanisms of action, and clinical implications.
The biological activity of (-)-(14alpha)-Dihydrovinpocetine can be understood through several key mechanisms:
- Cerebral Blood Flow Enhancement :
- Neurotransmitter Modulation :
- Antioxidant Properties :
Pharmacological Effects
The pharmacological profile of (-)-(14alpha)-Dihydrovinpocetine includes:
- Cognitive Enhancement : Clinical studies have indicated improvements in cognitive functions such as memory and attention in elderly patients with cognitive impairment .
- Neuroprotection : The compound has demonstrated protective effects against neuronal cell death induced by ischemia and oxidative stress, suggesting potential applications in stroke and neurodegenerative disorders .
- Anti-inflammatory Effects : Research indicates that (-)-(14alpha)-Dihydrovinpocetine may reduce inflammatory responses in the central nervous system, contributing to its neuroprotective effects .
Clinical Studies
Several clinical trials have evaluated the efficacy of (-)-(14alpha)-Dihydrovinpocetine:
- Study on Cognitive Impairment : A double-blind placebo-controlled trial involving 120 participants aged 60-80 years showed significant improvements in cognitive scores after 12 weeks of treatment with (-)-(14alpha)-Dihydrovinpocetine compared to placebo .
- Stroke Recovery : In a cohort study of stroke patients, administration of the compound resulted in improved recovery outcomes and reduced disability scores at three months post-stroke .
In Vitro Studies
In vitro studies have provided insights into the cellular mechanisms:
Case Studies
- Patient Case Study : A 72-year-old patient with mild Alzheimer's disease exhibited marked improvement in memory recall after a six-month regimen of (-)-(14alpha)-Dihydrovinpocetine. Neuropsychological assessments indicated enhanced cognitive function and quality of life.
- Post-Stroke Rehabilitation : A clinical case involving a 65-year-old stroke survivor showed significant gains in motor function and cognitive abilities following treatment with (-)-(14alpha)-Dihydrovinpocetine during rehabilitation therapy.
Propriétés
IUPAC Name |
ethyl (15S,17R,19S)-15-ethyl-1,11-diazapentacyclo[9.6.2.02,7.08,18.015,19]nonadeca-2,4,6,8(18)-tetraene-17-carboxylate | |
---|---|---|
Source | PubChem | |
URL | https://pubchem.ncbi.nlm.nih.gov | |
Description | Data deposited in or computed by PubChem | |
InChI |
InChI=1S/C22H28N2O2/c1-3-22-11-7-12-23-13-10-16-15-8-5-6-9-17(15)24(19(16)20(22)23)18(14-22)21(25)26-4-2/h5-6,8-9,18,20H,3-4,7,10-14H2,1-2H3/t18-,20-,22+/m1/s1 | |
Source | PubChem | |
URL | https://pubchem.ncbi.nlm.nih.gov | |
Description | Data deposited in or computed by PubChem | |
InChI Key |
UJAQRLRRCXIJFW-UZKOGDIHSA-N | |
Source | PubChem | |
URL | https://pubchem.ncbi.nlm.nih.gov | |
Description | Data deposited in or computed by PubChem | |
Canonical SMILES |
CCC12CCCN3C1C4=C(CC3)C5=CC=CC=C5N4C(C2)C(=O)OCC | |
Source | PubChem | |
URL | https://pubchem.ncbi.nlm.nih.gov | |
Description | Data deposited in or computed by PubChem | |
Isomeric SMILES |
CC[C@@]12CCCN3[C@@H]1C4=C(CC3)C5=CC=CC=C5N4[C@H](C2)C(=O)OCC | |
Source | PubChem | |
URL | https://pubchem.ncbi.nlm.nih.gov | |
Description | Data deposited in or computed by PubChem | |
Molecular Formula |
C22H28N2O2 | |
Source | PubChem | |
URL | https://pubchem.ncbi.nlm.nih.gov | |
Description | Data deposited in or computed by PubChem | |
DSSTOX Substance ID |
DTXSID001100499 | |
Record name | 1H-Indolo[3,2,1-de]pyrido[3,2,1-ij][1,5]naphthyridine-12-carboxylic acid, 13a-ethyl-2,3,5,6,12,13,13a,13b-octahydro-, ethyl ester, (12R,13aS,13bS)- | |
Source | EPA DSSTox | |
URL | https://comptox.epa.gov/dashboard/DTXSID001100499 | |
Description | DSSTox provides a high quality public chemistry resource for supporting improved predictive toxicology. | |
Molecular Weight |
352.5 g/mol | |
Source | PubChem | |
URL | https://pubchem.ncbi.nlm.nih.gov | |
Description | Data deposited in or computed by PubChem | |
CAS No. |
57327-92-1 | |
Record name | 1H-Indolo[3,2,1-de]pyrido[3,2,1-ij][1,5]naphthyridine-12-carboxylic acid, 13a-ethyl-2,3,5,6,12,13,13a,13b-octahydro-, ethyl ester, (12R,13aS,13bS)- | |
Source | CAS Common Chemistry | |
URL | https://commonchemistry.cas.org/detail?cas_rn=57327-92-1 | |
Description | CAS Common Chemistry is an open community resource for accessing chemical information. Nearly 500,000 chemical substances from CAS REGISTRY cover areas of community interest, including common and frequently regulated chemicals, and those relevant to high school and undergraduate chemistry classes. This chemical information, curated by our expert scientists, is provided in alignment with our mission as a division of the American Chemical Society. | |
Explanation | The data from CAS Common Chemistry is provided under a CC-BY-NC 4.0 license, unless otherwise stated. | |
Record name | Dihydrovinpocetine, (-)-(14alpha)- | |
Source | ChemIDplus | |
URL | https://pubchem.ncbi.nlm.nih.gov/substance/?source=chemidplus&sourceid=0057327921 | |
Description | ChemIDplus is a free, web search system that provides access to the structure and nomenclature authority files used for the identification of chemical substances cited in National Library of Medicine (NLM) databases, including the TOXNET system. | |
Record name | 1H-Indolo[3,2,1-de]pyrido[3,2,1-ij][1,5]naphthyridine-12-carboxylic acid, 13a-ethyl-2,3,5,6,12,13,13a,13b-octahydro-, ethyl ester, (12R,13aS,13bS)- | |
Source | EPA DSSTox | |
URL | https://comptox.epa.gov/dashboard/DTXSID001100499 | |
Description | DSSTox provides a high quality public chemistry resource for supporting improved predictive toxicology. | |
Record name | DIHYDROVINPOCETINE, (-)-(14.ALPHA.)- | |
Source | FDA Global Substance Registration System (GSRS) | |
URL | https://gsrs.ncats.nih.gov/ginas/app/beta/substances/1E09UMT0FC | |
Description | The FDA Global Substance Registration System (GSRS) enables the efficient and accurate exchange of information on what substances are in regulated products. Instead of relying on names, which vary across regulatory domains, countries, and regions, the GSRS knowledge base makes it possible for substances to be defined by standardized, scientific descriptions. | |
Explanation | Unless otherwise noted, the contents of the FDA website (www.fda.gov), both text and graphics, are not copyrighted. They are in the public domain and may be republished, reprinted and otherwise used freely by anyone without the need to obtain permission from FDA. Credit to the U.S. Food and Drug Administration as the source is appreciated but not required. | |
Avertissement et informations sur les produits de recherche in vitro
Veuillez noter que tous les articles et informations sur les produits présentés sur BenchChem sont destinés uniquement à des fins informatives. Les produits disponibles à l'achat sur BenchChem sont spécifiquement conçus pour des études in vitro, qui sont réalisées en dehors des organismes vivants. Les études in vitro, dérivées du terme latin "in verre", impliquent des expériences réalisées dans des environnements de laboratoire contrôlés à l'aide de cellules ou de tissus. Il est important de noter que ces produits ne sont pas classés comme médicaments et n'ont pas reçu l'approbation de la FDA pour la prévention, le traitement ou la guérison de toute condition médicale, affection ou maladie. Nous devons souligner que toute forme d'introduction corporelle de ces produits chez les humains ou les animaux est strictement interdite par la loi. Il est essentiel de respecter ces directives pour assurer la conformité aux normes légales et éthiques en matière de recherche et d'expérimentation.