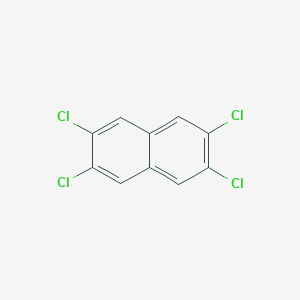
2,3,6,7-Tétrachloronaphtalène
Vue d'ensemble
Description
2,3,6,7-Tetrachloronaphthalene is a polychlorinated naphthalene, a class of compounds derived from naphthalene through the substitution of hydrogen atoms with chlorine atoms. This compound is characterized by the presence of four chlorine atoms at the 2, 3, 6, and 7 positions on the naphthalene ring. It has the molecular formula C10H4Cl4 and a molecular weight of 265.95 g/mol .
Applications De Recherche Scientifique
2,3,6,7-Tetrachloronaphthalene has several scientific research applications, including:
Chemistry: Used as a reference standard in analytical chemistry for the study of polychlorinated naphthalenes.
Biology: Investigated for its effects on biological systems, including its potential as an environmental pollutant.
Medicine: Studied for its toxicological properties and potential health effects.
Industry: Used in the production of insulating coatings for electrical wires and other industrial applications
Méthodes De Préparation
Synthetic Routes and Reaction Conditions: 2,3,6,7-Tetrachloronaphthalene can be synthesized through the chlorination of naphthalene. The process involves the reaction of naphthalene with chlorine gas in the presence of a catalyst, such as ferric chloride (FeCl3), at elevated temperatures. The reaction proceeds through a series of electrophilic aromatic substitution steps, resulting in the formation of the tetrachlorinated product .
Industrial Production Methods: Industrial production of 2,3,6,7-Tetrachloronaphthalene typically involves large-scale chlorination processes. The reaction conditions are carefully controlled to ensure the selective chlorination of naphthalene at the desired positions. The product is then purified through distillation or recrystallization to obtain the desired compound in high purity .
Analyse Des Réactions Chimiques
Types of Reactions: 2,3,6,7-Tetrachloronaphthalene undergoes various chemical reactions, including:
Oxidation: The compound can be oxidized to form chlorinated naphthoquinones.
Reduction: Reduction reactions can lead to the formation of partially dechlorinated naphthalenes.
Substitution: Electrophilic substitution reactions can introduce other functional groups onto the naphthalene ring.
Common Reagents and Conditions:
Oxidation: Reagents such as potassium permanganate (KMnO4) or chromium trioxide (CrO3) in acidic conditions.
Reduction: Reagents like sodium borohydride (NaBH4) or lithium aluminum hydride (LiAlH4).
Substitution: Reagents such as nitric acid (HNO3) or sulfuric acid (H2SO4) for nitration and sulfonation reactions.
Major Products:
Oxidation: Chlorinated naphthoquinones.
Reduction: Partially dechlorinated naphthalenes.
Substitution: Nitrated or sulfonated derivatives of 2,3,6,7-Tetrachloronaphthalene.
Mécanisme D'action
The mechanism of action of 2,3,6,7-Tetrachloronaphthalene involves its interaction with cellular components, leading to various biological effects. The compound can bind to and activate certain receptors, such as the aryl hydrocarbon receptor (AhR), which regulates the expression of genes involved in xenobiotic metabolism. This interaction can lead to the induction of enzymes responsible for the detoxification and elimination of the compound from the body .
Comparaison Avec Des Composés Similaires
- 1,2,3,4-Tetrachloronaphthalene
- 1,2,3,5-Tetrachloronaphthalene
- 1,2,3,6-Tetrachloronaphthalene
- 1,2,3,7-Tetrachloronaphthalene
Comparison: 2,3,6,7-Tetrachloronaphthalene is unique due to the specific positions of the chlorine atoms on the naphthalene ring. This unique arrangement influences its chemical reactivity, physical properties, and biological effects. For example, 1,2,3,4-Tetrachloronaphthalene is more polar than 2,3,6,7-Tetrachloronaphthalene, leading to differences in solubility and reactivity .
Propriétés
IUPAC Name |
2,3,6,7-tetrachloronaphthalene | |
---|---|---|
Source | PubChem | |
URL | https://pubchem.ncbi.nlm.nih.gov | |
Description | Data deposited in or computed by PubChem | |
InChI |
InChI=1S/C10H4Cl4/c11-7-1-5-2-9(13)10(14)4-6(5)3-8(7)12/h1-4H | |
Source | PubChem | |
URL | https://pubchem.ncbi.nlm.nih.gov | |
Description | Data deposited in or computed by PubChem | |
InChI Key |
XTTLUUBHRXWFSZ-UHFFFAOYSA-N | |
Source | PubChem | |
URL | https://pubchem.ncbi.nlm.nih.gov | |
Description | Data deposited in or computed by PubChem | |
Canonical SMILES |
C1=C2C=C(C(=CC2=CC(=C1Cl)Cl)Cl)Cl | |
Source | PubChem | |
URL | https://pubchem.ncbi.nlm.nih.gov | |
Description | Data deposited in or computed by PubChem | |
Molecular Formula |
C10H4Cl4 | |
Source | PubChem | |
URL | https://pubchem.ncbi.nlm.nih.gov | |
Description | Data deposited in or computed by PubChem | |
DSSTOX Substance ID |
DTXSID50188145 | |
Record name | 2,3,6,7-Tetrachloronaphthalene | |
Source | EPA DSSTox | |
URL | https://comptox.epa.gov/dashboard/DTXSID50188145 | |
Description | DSSTox provides a high quality public chemistry resource for supporting improved predictive toxicology. | |
Molecular Weight |
265.9 g/mol | |
Source | PubChem | |
URL | https://pubchem.ncbi.nlm.nih.gov | |
Description | Data deposited in or computed by PubChem | |
CAS No. |
34588-40-4 | |
Record name | 2,3,6,7-Tetrachloronaphthalene | |
Source | CAS Common Chemistry | |
URL | https://commonchemistry.cas.org/detail?cas_rn=34588-40-4 | |
Description | CAS Common Chemistry is an open community resource for accessing chemical information. Nearly 500,000 chemical substances from CAS REGISTRY cover areas of community interest, including common and frequently regulated chemicals, and those relevant to high school and undergraduate chemistry classes. This chemical information, curated by our expert scientists, is provided in alignment with our mission as a division of the American Chemical Society. | |
Explanation | The data from CAS Common Chemistry is provided under a CC-BY-NC 4.0 license, unless otherwise stated. | |
Record name | Naphthalene, 2,3,6,7-tetrachloro- | |
Source | ChemIDplus | |
URL | https://pubchem.ncbi.nlm.nih.gov/substance/?source=chemidplus&sourceid=0034588404 | |
Description | ChemIDplus is a free, web search system that provides access to the structure and nomenclature authority files used for the identification of chemical substances cited in National Library of Medicine (NLM) databases, including the TOXNET system. | |
Record name | 2,3,6,7-Tetrachloronaphthalene | |
Source | EPA DSSTox | |
URL | https://comptox.epa.gov/dashboard/DTXSID50188145 | |
Description | DSSTox provides a high quality public chemistry resource for supporting improved predictive toxicology. | |
Retrosynthesis Analysis
AI-Powered Synthesis Planning: Our tool employs the Template_relevance Pistachio, Template_relevance Bkms_metabolic, Template_relevance Pistachio_ringbreaker, Template_relevance Reaxys, Template_relevance Reaxys_biocatalysis model, leveraging a vast database of chemical reactions to predict feasible synthetic routes.
One-Step Synthesis Focus: Specifically designed for one-step synthesis, it provides concise and direct routes for your target compounds, streamlining the synthesis process.
Accurate Predictions: Utilizing the extensive PISTACHIO, BKMS_METABOLIC, PISTACHIO_RINGBREAKER, REAXYS, REAXYS_BIOCATALYSIS database, our tool offers high-accuracy predictions, reflecting the latest in chemical research and data.
Strategy Settings
Precursor scoring | Relevance Heuristic |
---|---|
Min. plausibility | 0.01 |
Model | Template_relevance |
Template Set | Pistachio/Bkms_metabolic/Pistachio_ringbreaker/Reaxys/Reaxys_biocatalysis |
Top-N result to add to graph | 6 |
Feasible Synthetic Routes
Q1: What are the magnetic properties of coordination polymers incorporating 2,3,6,7-tetrachloronaphthalene-1,4,5,8-tetrathiolato ligands?
A1: Research has shown that nickel and cobalt coordination polymers containing 2,3,6,7-tetrachloronaphthalene-1,4,5,8-tetrathiolato ligands exhibit interesting magnetic behavior. Specifically, a nickel coordination polymer (complex 7 in the study) displayed ferromagnetic properties below 20 K. [] This ferromagnetism was confirmed through temperature-dependent magnetic susceptibility measurements, magnetization behavior analysis, and heat capacity studies. Furthermore, the complex exhibited a coercive field of 1200 Oe at 4.4 K, as evidenced by its hysteresis loop. [] Similar magnetic characteristics were also observed in analogous cobalt complexes. [] This highlights the potential of these types of compounds in the field of molecular magnetism.
Q2: How is 2,3,6,7-Tetrachloronaphthalene synthesized?
A2: 2,3,6,7-Tetrachloronaphthalene can be synthesized through a cycloaddition reaction involving 4,5-dichloro-o-quinodimethane. [] This approach provides a viable route to obtain 2,3,6,7-tetrasubstituted naphthalene derivatives. []
Avertissement et informations sur les produits de recherche in vitro
Veuillez noter que tous les articles et informations sur les produits présentés sur BenchChem sont destinés uniquement à des fins informatives. Les produits disponibles à l'achat sur BenchChem sont spécifiquement conçus pour des études in vitro, qui sont réalisées en dehors des organismes vivants. Les études in vitro, dérivées du terme latin "in verre", impliquent des expériences réalisées dans des environnements de laboratoire contrôlés à l'aide de cellules ou de tissus. Il est important de noter que ces produits ne sont pas classés comme médicaments et n'ont pas reçu l'approbation de la FDA pour la prévention, le traitement ou la guérison de toute condition médicale, affection ou maladie. Nous devons souligner que toute forme d'introduction corporelle de ces produits chez les humains ou les animaux est strictement interdite par la loi. Il est essentiel de respecter ces directives pour assurer la conformité aux normes légales et éthiques en matière de recherche et d'expérimentation.