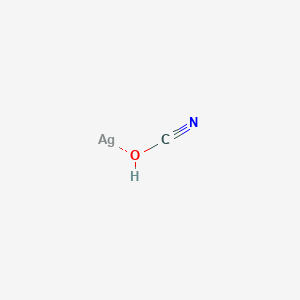
Silver cyanate
Vue d'ensemble
Description
Silver cyanate is an inorganic compound composed of silver, carbon, oxygen, and nitrogen atoms . It is a beige to gray powder and is used as a chemosterilant in adult house flies . It is also used as a reagent to prepare certain derivatives which have potential anti-human immunodeficiency virus (HIV) and antitumor activity . Additionally, it is used as a silver plating solution in the electroplating process .
Synthesis Analysis
Silver cyanate can be synthesized by the reaction of potassium cyanate with silver nitrate in aqueous solution, from which it precipitates as a solid . The reaction is given by: AgNO3 + KNCO → Ag(NCO) + K+ + NO−3 . Alternatively, the reaction AgNO3 + CO(NH2)2 → AgNCO + NH4NO3 may be used .
Molecular Structure Analysis
Silver cyanate crystallizes in the monoclinic crystal system in space group P 2 1 / m with parameters a = 547.3 pm, b = 637.2 pm, c = 341.6 pm, and β = 91° . Each unit cell contains two cyanate ions and two silver ions . The silver ions are each equidistant from two nitrogen atoms forming a straight N–Ag–N group . The nitrogen atoms are each coordinated to two silver atoms, so that there are zigzag chains of alternating silver and nitrogen atoms going in the direction of the monoclinic “b” axis, with the cyanate ions perpendicular to that axis .
Chemical Reactions Analysis
Silver cyanate reacts with nitric acid to form silver nitrate, carbon dioxide, and ammonium nitrate . The reaction is given by: AgNCO + 2 HNO3 + H2O → AgNO3 + CO2 + NH4NO3 .
Physical And Chemical Properties Analysis
Silver cyanate is a colorless compound with a molecular weight of 150.893 g/mol . It is soluble in ammonia, nitric acid, potassium cyanide, and ammonium hydroxide, but insoluble in alcohol and dilute acids .
Applications De Recherche Scientifique
Organic Compound Synthesis
Silver cyanate is a valuable tool in organic compound synthesis . It is used to prepare 1-(3-thianyl)uracil and 9-(3-thianyl)adenine derivatives .
Oxidizing Agent
The silver ion within the silver cyanate molecule functions as a Lewis acid, making silver cyanate an effective oxidizing agent .
Catalyst for Organic Reactions
The cyanate ion in silver cyanate acts as a Lewis base, contributing to its catalytic properties . It is used as a catalyst in organic reactions .
Chemosterilant
Silver cyanate is used as a chemosterilant in adult house flies . This application is particularly useful in pest control.
Anti-HIV and Antitumor Activity
Silver cyanate is used to prepare derivatives that have potential anti-human immunodeficiency virus (HIV) and antitumor activity .
Silver Plating Solution
In the electroplating industry, silver cyanate is used as a silver plating solution .
Research in Radiochemistry
Silver cyanate has been used in the efficient and practical radiosynthesis of [11C]temozolomide .
Synthesis of Artificial Nucleic Acid
Silver cyanate has been used in the synthesis of an artificial nucleic acid bearing an oxanorbornane scaffold .
Safety and Hazards
Silver cyanate is considered hazardous according to the 2012 OSHA Hazard Communication Standard . It has been associated with acute oral toxicity, acute dermal toxicity, and acute inhalation toxicity. It can cause skin corrosion/irritation and serious eye damage/eye irritation . It is recommended to avoid breathing mist, gas, or vapors, avoid contact with skin and eyes, and use personal protective equipment when handling silver cyanate .
Orientations Futures
The Silver Cyanate Market size is projected to reach approximately USD XX.X billion by 2031, up from USD XX.X billion in 2023 . This growth is expected to occur at a CAGR of XX.X% during the forecast period from 2024 to 2031 . Technological advancements have led to the development of innovative Silver Cyanate solutions, meeting diverse consumer needs across industries . Increasing awareness of Silver Cyanate benefits, coupled with growing environmental concerns, is fueling market expansion .
Mécanisme D'action
Target of Action
Silver cyanate, a cyanate salt of silver , primarily targets nitrogen atoms in its environment. In its crystalline structure, each silver ion is equidistant from two nitrogen atoms, forming a straight N–Ag–N group . This suggests that nitrogen-containing compounds could be potential targets for silver cyanate.
Mode of Action
The mode of action of silver cyanate involves its interaction with nitrogen atoms. The silver ions in silver cyanate are each coordinated to two nitrogen atoms, resulting in zigzag chains of alternating silver and nitrogen atoms . This unique structure allows silver cyanate to interact with nitrogen-containing compounds in its environment.
Biochemical Pathways
Cyanate, a component of silver cyanate, is known to be toxic to all organisms . Cyanase, an enzyme found in many organisms, converts cyanate to CO2 and NH3 in a bicarbonate-dependent reaction . This suggests that silver cyanate may potentially interfere with this detoxification pathway.
Pharmacokinetics
It’s known that silver cyanate is soluble in ammonia, nitric acid, potassium cyanide, and ammonium hydroxide, but insoluble in alcohol and dilute acids . This solubility profile may influence its bioavailability and distribution within the body.
Action Environment
The action of silver cyanate can be influenced by environmental factors. For instance, its solubility varies depending on the presence of certain substances, such as ammonia or nitric acid . This suggests that the action, efficacy, and stability of silver cyanate could be affected by the chemical composition of its environment.
Propriétés
IUPAC Name |
cyanic acid;silver | |
---|---|---|
Source | PubChem | |
URL | https://pubchem.ncbi.nlm.nih.gov | |
Description | Data deposited in or computed by PubChem | |
InChI |
InChI=1S/CHNO.Ag/c2-1-3;/h3H; | |
Source | PubChem | |
URL | https://pubchem.ncbi.nlm.nih.gov | |
Description | Data deposited in or computed by PubChem | |
InChI Key |
VAQVDZZAAMAZAO-UHFFFAOYSA-N | |
Source | PubChem | |
URL | https://pubchem.ncbi.nlm.nih.gov | |
Description | Data deposited in or computed by PubChem | |
Canonical SMILES |
C(#N)O.[Ag] | |
Source | PubChem | |
URL | https://pubchem.ncbi.nlm.nih.gov | |
Description | Data deposited in or computed by PubChem | |
Molecular Formula |
CHAgNO | |
Source | PubChem | |
URL | https://pubchem.ncbi.nlm.nih.gov | |
Description | Data deposited in or computed by PubChem | |
Molecular Weight |
150.893 g/mol | |
Source | PubChem | |
URL | https://pubchem.ncbi.nlm.nih.gov | |
Description | Data deposited in or computed by PubChem | |
Physical Description |
White odorless powder; Soluble in water; [Alfa Aesar MSDS] | |
Record name | Silver cyanate | |
Source | Haz-Map, Information on Hazardous Chemicals and Occupational Diseases | |
URL | https://haz-map.com/Agents/21687 | |
Description | Haz-Map® is an occupational health database designed for health and safety professionals and for consumers seeking information about the adverse effects of workplace exposures to chemical and biological agents. | |
Explanation | Copyright (c) 2022 Haz-Map(R). All rights reserved. Unless otherwise indicated, all materials from Haz-Map are copyrighted by Haz-Map(R). No part of these materials, either text or image may be used for any purpose other than for personal use. Therefore, reproduction, modification, storage in a retrieval system or retransmission, in any form or by any means, electronic, mechanical or otherwise, for reasons other than personal use, is strictly prohibited without prior written permission. | |
Product Name |
Silver cyanate | |
CAS RN |
3315-16-0 | |
Record name | Silver cyanate | |
Source | CAS Common Chemistry | |
URL | https://commonchemistry.cas.org/detail?cas_rn=3315-16-0 | |
Description | CAS Common Chemistry is an open community resource for accessing chemical information. Nearly 500,000 chemical substances from CAS REGISTRY cover areas of community interest, including common and frequently regulated chemicals, and those relevant to high school and undergraduate chemistry classes. This chemical information, curated by our expert scientists, is provided in alignment with our mission as a division of the American Chemical Society. | |
Explanation | The data from CAS Common Chemistry is provided under a CC-BY-NC 4.0 license, unless otherwise stated. | |
Record name | Cyanic acid, silver(1+) salt (1:1) | |
Source | EPA Chemicals under the TSCA | |
URL | https://www.epa.gov/chemicals-under-tsca | |
Description | EPA Chemicals under the Toxic Substances Control Act (TSCA) collection contains information on chemicals and their regulations under TSCA, including non-confidential content from the TSCA Chemical Substance Inventory and Chemical Data Reporting. | |
Record name | Silver cyanate | |
Source | European Chemicals Agency (ECHA) | |
URL | https://echa.europa.eu/substance-information/-/substanceinfo/100.020.007 | |
Description | The European Chemicals Agency (ECHA) is an agency of the European Union which is the driving force among regulatory authorities in implementing the EU's groundbreaking chemicals legislation for the benefit of human health and the environment as well as for innovation and competitiveness. | |
Explanation | Use of the information, documents and data from the ECHA website is subject to the terms and conditions of this Legal Notice, and subject to other binding limitations provided for under applicable law, the information, documents and data made available on the ECHA website may be reproduced, distributed and/or used, totally or in part, for non-commercial purposes provided that ECHA is acknowledged as the source: "Source: European Chemicals Agency, http://echa.europa.eu/". Such acknowledgement must be included in each copy of the material. ECHA permits and encourages organisations and individuals to create links to the ECHA website under the following cumulative conditions: Links can only be made to webpages that provide a link to the Legal Notice page. | |
Retrosynthesis Analysis
AI-Powered Synthesis Planning: Our tool employs the Template_relevance Pistachio, Template_relevance Bkms_metabolic, Template_relevance Pistachio_ringbreaker, Template_relevance Reaxys, Template_relevance Reaxys_biocatalysis model, leveraging a vast database of chemical reactions to predict feasible synthetic routes.
One-Step Synthesis Focus: Specifically designed for one-step synthesis, it provides concise and direct routes for your target compounds, streamlining the synthesis process.
Accurate Predictions: Utilizing the extensive PISTACHIO, BKMS_METABOLIC, PISTACHIO_RINGBREAKER, REAXYS, REAXYS_BIOCATALYSIS database, our tool offers high-accuracy predictions, reflecting the latest in chemical research and data.
Strategy Settings
Precursor scoring | Relevance Heuristic |
---|---|
Min. plausibility | 0.01 |
Model | Template_relevance |
Template Set | Pistachio/Bkms_metabolic/Pistachio_ringbreaker/Reaxys/Reaxys_biocatalysis |
Top-N result to add to graph | 6 |
Feasible Synthetic Routes
Avertissement et informations sur les produits de recherche in vitro
Veuillez noter que tous les articles et informations sur les produits présentés sur BenchChem sont destinés uniquement à des fins informatives. Les produits disponibles à l'achat sur BenchChem sont spécifiquement conçus pour des études in vitro, qui sont réalisées en dehors des organismes vivants. Les études in vitro, dérivées du terme latin "in verre", impliquent des expériences réalisées dans des environnements de laboratoire contrôlés à l'aide de cellules ou de tissus. Il est important de noter que ces produits ne sont pas classés comme médicaments et n'ont pas reçu l'approbation de la FDA pour la prévention, le traitement ou la guérison de toute condition médicale, affection ou maladie. Nous devons souligner que toute forme d'introduction corporelle de ces produits chez les humains ou les animaux est strictement interdite par la loi. Il est essentiel de respecter ces directives pour assurer la conformité aux normes légales et éthiques en matière de recherche et d'expérimentation.