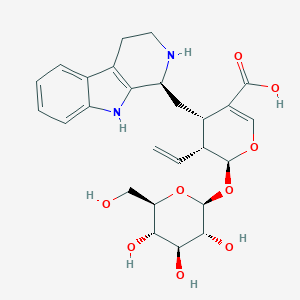
Acide strictosidinique
Vue d'ensemble
Description
L’omiloxetine, également connue sous le nom d’omiloextinum ou d’omiloxetino, est un inhibiteur sélectif de la recapture de la sérotonine (ISRS) qui a été développé comme un antidépresseur potentiel. Elle a été initialement étudiée par la société pharmaceutique espagnole Ferrer Internacional. Malgré ses résultats précliniques prometteurs, le développement de l’omiloxetine a été interrompu en 2005 .
Applications De Recherche Scientifique
Chemistry: As a selective serotonin reuptake inhibitor, omiloxetine has been used in studies to understand the mechanisms of SSRIs and their interactions with serotonin transporters.
Biology: The compound has been used in research to explore its effects on serotonin levels in the brain and its potential therapeutic effects on mood disorders.
Medicine: Although its development was discontinued, omiloxetine was initially investigated as a potential treatment for depression and other mood disorders.
Industry: The compound’s unique chemical structure has made it a subject of interest in the development of new SSRIs and other related compounds.
Mécanisme D'action
L’omiloxetine exerce ses effets en inhibant sélectivement la recapture de la sérotonine dans le cerveau. Cela conduit à une augmentation des niveaux de sérotonine dans la fente synaptique, ce qui améliore la signalisation de la sérotonine et améliore l’humeur. La principale cible moléculaire de l’omiloxetine est le transporteur de la sérotonine (SERT), qui est responsable de la recapture de la sérotonine de la fente synaptique vers le neurone présynaptique .
Analyse Biochimique
Biochemical Properties
Strictosidinic acid interacts with the enzymes involved in the biosynthesis of 5-HT, a neurotransmitter . By inhibiting these enzymes, strictosidinic acid effectively reduces the levels of 5-HT . The nature of these interactions is inhibitory, leading to a decrease in the production of 5-HT .
Cellular Effects
Strictosidinic acid influences cell function by modulating the levels of 5-HT . As 5-HT plays a crucial role in various cellular processes, including cell signaling pathways and gene expression, the reduction in 5-HT levels caused by strictosidinic acid can have significant effects on cellular function .
Molecular Mechanism
The molecular mechanism of action of strictosidinic acid involves binding interactions with the precursor enzymes of 5-HT biosynthesis . By inhibiting these enzymes, strictosidinic acid prevents the conversion of precursor molecules into 5-HT, thereby reducing the overall levels of 5-HT .
Dosage Effects in Animal Models
In animal models, strictosidinic acid has been shown to have peripheral analgesic and antipyretic activities
Metabolic Pathways
Strictosidinic acid is involved in the metabolic pathway of 5-HT biosynthesis . It interacts with the precursor enzymes of this pathway, inhibiting their activity and thereby reducing the production of 5-HT .
Méthodes De Préparation
La synthèse de l’omiloxetine implique plusieurs étapes clés :
Formation du cycle pipéridine : La synthèse commence par la formation du cycle pipéridine, qui est un élément crucial de la molécule.
Introduction de groupes fluorophényles :
Formation du groupe benzodioxole : Le groupe benzodioxole est ensuite introduit, ce qui est essentiel pour l’activité du composé en tant qu’ISRS.
Assemblage final : L’étape finale consiste à coupler les intermédiaires formés précédemment pour produire la molécule finale d’omiloxetine.
Les méthodes de production industrielle de l’omiloxetine impliqueraient probablement l’optimisation de ces voies de synthèse pour garantir un rendement et une pureté élevés, ainsi que la rentabilité et la possibilité de mise à l’échelle.
Analyse Des Réactions Chimiques
L’omiloxetine subit plusieurs types de réactions chimiques :
Oxydation : L’omiloxetine peut être oxydée dans des conditions spécifiques, conduisant à la formation de divers produits d’oxydation.
Réduction : Le composé peut également subir des réactions de réduction, bien que celles-ci soient moins courantes.
Substitution : L’omiloxetine peut participer à des réactions de substitution, en particulier impliquant les groupes fluorophényles.
Les réactifs et les conditions courantes utilisés dans ces réactions comprennent les agents oxydants comme le permanganate de potassium pour l’oxydation, les agents réducteurs comme l’hydrure de lithium et d’aluminium pour la réduction et les nucléophiles pour les réactions de substitution. Les principaux produits formés à partir de ces réactions dépendent des conditions et des réactifs spécifiques utilisés.
4. Applications de la Recherche Scientifique
Chimie : En tant qu’inhibiteur sélectif de la recapture de la sérotonine, l’omiloxetine a été utilisée dans des études pour comprendre les mécanismes des ISRS et leurs interactions avec les transporteurs de la sérotonine.
Biologie : Le composé a été utilisé dans la recherche pour explorer ses effets sur les niveaux de sérotonine dans le cerveau et ses effets thérapeutiques potentiels sur les troubles de l’humeur.
Médecine : Bien que son développement ait été interrompu, l’omiloxetine a été initialement étudiée comme un traitement potentiel de la dépression et d’autres troubles de l’humeur.
Industrie : La structure chimique unique du composé a fait de lui un sujet d’intérêt dans le développement de nouveaux ISRS et d’autres composés apparentés.
Comparaison Avec Des Composés Similaires
L’omiloxetine est similaire à d’autres inhibiteurs sélectifs de la recapture de la sérotonine comme la fluoxétine, la sertraline et la paroxétine. Elle présente des caractéristiques structurelles uniques, telles que la présence d’un groupe benzodioxole et des substitutions fluorophényles spécifiques, qui la différencient des autres ISRS. Ces caractéristiques uniques contribuent à son affinité de liaison spécifique et à sa sélectivité pour le transporteur de la sérotonine.
Composés Similaires
- Fluoxétine
- Sertraline
- Paroxétine
- Citalopram
- Escitalopram
Ces composés partagent un mécanisme d’action commun, mais diffèrent par leurs structures chimiques, leur pharmacocinétique et leurs profils d’effets secondaires.
Activité Biologique
Strictosidinic acid, an alkaloid derived from the Camptotheca acuminata plant, has garnered attention due to its significant biological properties. This article delves into the compound's biological activity, exploring its roles in various biochemical pathways, potential therapeutic effects, and the implications of its structural isomers.
Overview of Strictosidinic Acid
Strictosidinic acid is a precursor in the biosynthesis of camptothecin (CPT), a potent anticancer agent. It is synthesized through a complex metabolic pathway involving tryptamine and secologanin. The compound exists in multiple isomeric forms, which can influence its biological activity and pharmacological potential.
Metabolic Pathways
Strictosidinic acid plays a crucial role in the biosynthesis of several important metabolites:
- Camptothecin Pathway : Strictosidinic acid and strictosamide are identified as critical bio-precursors for CPT in C. acuminata. Studies have shown that elicitors such as methyl jasmonate (MeJa) significantly enhance CPT levels by promoting the carbon metabolism and secondary metabolic pathways involved in strictosidinic acid synthesis .
- Isomer Diversity : The presence of diastereomers of strictosidinic acid has been documented, with variations in their accumulation across different plant tissues. For instance, the major accumulation sites for strictosidinic acid were found to be in the shoot apex and young leaves, while mature leaves and roots showed lower levels .
Central Nervous System Effects
Research indicates that strictosidinic acid exhibits promising effects on the central nervous system (CNS). A study involving rat models demonstrated that administration of strictosidinic acid resulted in significant alterations in monoamine levels within the striatum, suggesting potential neuropharmacological applications . This finding warrants further investigation into its mechanisms and therapeutic uses.
Anticancer Properties
The role of strictosidinic acid as a precursor for camptothecin underscores its potential anticancer activity. Camptothecin has been extensively studied for its ability to inhibit DNA topoisomerase I, leading to apoptosis in cancer cells. Thus, enhancing strictosidinic acid production through biotechnological methods could increase camptothecin yield and efficacy .
Case Studies and Research Findings
- Elicitor-Induced Biosynthesis :
-
Tissue Distribution Analysis :
- In a comprehensive metabolite profiling study, strictosidinic acid was found to be present in multiple isomeric forms across different tissues of C. acuminata. The study utilized UHPLC/MS techniques to analyze tissue samples, revealing distinct accumulation patterns that could influence pharmacological outcomes .
Data Tables
Study | Finding | Methodology | Implication |
---|---|---|---|
Sadre et al., 2016 | Strictosidinic acid enhances CPT biosynthesis | Elicitor treatment (MeJa) | Potential for increased anticancer drug production |
Pu et al., 2020 | Multiple isomers identified in plant tissues | UHPLC/MS analysis | Variability in biological activity based on isomer type |
Zhang et al., 2021 | CNS effects observed post-administration | Rat model studies | Possible neuropharmacological applications |
Propriétés
IUPAC Name |
(2S,3R,4S)-3-ethenyl-4-[[(1S)-2,3,4,9-tetrahydro-1H-pyrido[3,4-b]indol-1-yl]methyl]-2-[(2S,3R,4S,5S,6R)-3,4,5-trihydroxy-6-(hydroxymethyl)oxan-2-yl]oxy-3,4-dihydro-2H-pyran-5-carboxylic acid | |
---|---|---|
Source | PubChem | |
URL | https://pubchem.ncbi.nlm.nih.gov | |
Description | Data deposited in or computed by PubChem | |
InChI |
InChI=1S/C26H32N2O9/c1-2-12-15(9-18-20-14(7-8-27-18)13-5-3-4-6-17(13)28-20)16(24(33)34)11-35-25(12)37-26-23(32)22(31)21(30)19(10-29)36-26/h2-6,11-12,15,18-19,21-23,25-32H,1,7-10H2,(H,33,34)/t12-,15+,18+,19-,21-,22+,23-,25+,26+/m1/s1 | |
Source | PubChem | |
URL | https://pubchem.ncbi.nlm.nih.gov | |
Description | Data deposited in or computed by PubChem | |
InChI Key |
CMMIVMFGFIBAGC-IUNANRIWSA-N | |
Source | PubChem | |
URL | https://pubchem.ncbi.nlm.nih.gov | |
Description | Data deposited in or computed by PubChem | |
Canonical SMILES |
C=CC1C(C(=COC1OC2C(C(C(C(O2)CO)O)O)O)C(=O)O)CC3C4=C(CCN3)C5=CC=CC=C5N4 | |
Source | PubChem | |
URL | https://pubchem.ncbi.nlm.nih.gov | |
Description | Data deposited in or computed by PubChem | |
Isomeric SMILES |
C=C[C@@H]1[C@@H](C(=CO[C@H]1O[C@H]2[C@@H]([C@H]([C@@H]([C@H](O2)CO)O)O)O)C(=O)O)C[C@H]3C4=C(CCN3)C5=CC=CC=C5N4 | |
Source | PubChem | |
URL | https://pubchem.ncbi.nlm.nih.gov | |
Description | Data deposited in or computed by PubChem | |
Molecular Formula |
C26H32N2O9 | |
Source | PubChem | |
URL | https://pubchem.ncbi.nlm.nih.gov | |
Description | Data deposited in or computed by PubChem | |
DSSTOX Substance ID |
DTXSID201315444 | |
Record name | Strictosidinic acid | |
Source | EPA DSSTox | |
URL | https://comptox.epa.gov/dashboard/DTXSID201315444 | |
Description | DSSTox provides a high quality public chemistry resource for supporting improved predictive toxicology. | |
Molecular Weight |
516.5 g/mol | |
Source | PubChem | |
URL | https://pubchem.ncbi.nlm.nih.gov | |
Description | Data deposited in or computed by PubChem | |
CAS No. |
150148-81-5 | |
Record name | Strictosidinic acid | |
Source | CAS Common Chemistry | |
URL | https://commonchemistry.cas.org/detail?cas_rn=150148-81-5 | |
Description | CAS Common Chemistry is an open community resource for accessing chemical information. Nearly 500,000 chemical substances from CAS REGISTRY cover areas of community interest, including common and frequently regulated chemicals, and those relevant to high school and undergraduate chemistry classes. This chemical information, curated by our expert scientists, is provided in alignment with our mission as a division of the American Chemical Society. | |
Explanation | The data from CAS Common Chemistry is provided under a CC-BY-NC 4.0 license, unless otherwise stated. | |
Record name | Strictosidinic acid | |
Source | EPA DSSTox | |
URL | https://comptox.epa.gov/dashboard/DTXSID201315444 | |
Description | DSSTox provides a high quality public chemistry resource for supporting improved predictive toxicology. | |
Retrosynthesis Analysis
AI-Powered Synthesis Planning: Our tool employs the Template_relevance Pistachio, Template_relevance Bkms_metabolic, Template_relevance Pistachio_ringbreaker, Template_relevance Reaxys, Template_relevance Reaxys_biocatalysis model, leveraging a vast database of chemical reactions to predict feasible synthetic routes.
One-Step Synthesis Focus: Specifically designed for one-step synthesis, it provides concise and direct routes for your target compounds, streamlining the synthesis process.
Accurate Predictions: Utilizing the extensive PISTACHIO, BKMS_METABOLIC, PISTACHIO_RINGBREAKER, REAXYS, REAXYS_BIOCATALYSIS database, our tool offers high-accuracy predictions, reflecting the latest in chemical research and data.
Strategy Settings
Precursor scoring | Relevance Heuristic |
---|---|
Min. plausibility | 0.01 |
Model | Template_relevance |
Template Set | Pistachio/Bkms_metabolic/Pistachio_ringbreaker/Reaxys/Reaxys_biocatalysis |
Top-N result to add to graph | 6 |
Feasible Synthetic Routes
Avertissement et informations sur les produits de recherche in vitro
Veuillez noter que tous les articles et informations sur les produits présentés sur BenchChem sont destinés uniquement à des fins informatives. Les produits disponibles à l'achat sur BenchChem sont spécifiquement conçus pour des études in vitro, qui sont réalisées en dehors des organismes vivants. Les études in vitro, dérivées du terme latin "in verre", impliquent des expériences réalisées dans des environnements de laboratoire contrôlés à l'aide de cellules ou de tissus. Il est important de noter que ces produits ne sont pas classés comme médicaments et n'ont pas reçu l'approbation de la FDA pour la prévention, le traitement ou la guérison de toute condition médicale, affection ou maladie. Nous devons souligner que toute forme d'introduction corporelle de ces produits chez les humains ou les animaux est strictement interdite par la loi. Il est essentiel de respecter ces directives pour assurer la conformité aux normes légales et éthiques en matière de recherche et d'expérimentation.