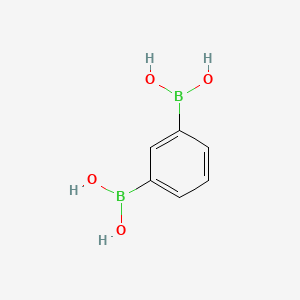
1,3-Benzenediboronic acid
Vue d'ensemble
Description
1,3-Benzenediboronic acid, also known as 1,3-diboronic acid, is a versatile and important organic compound used in a variety of scientific applications. It is a diboronic acid, which is an organic compound that contains two boron atoms linked to two oxygen atoms. It is a colorless, crystalline solid with a melting point of 171-173 °C. It is soluble in water and is used as a reagent in organic synthesis. This compound is a key building block in the synthesis of a wide range of organic molecules, including pharmaceuticals, polymers, and other materials. It is also used in a variety of biochemical and physiological studies, as well as in the development of new drugs.
Applications De Recherche Scientifique
Fluorescent Biosensing
- Research Focus : Surface engineering of carbon dots with benzenediboronic acid for fluorescent biosensing, specifically for glucose sensing in clinical diagnostics of diabetes. The benzenediboronic acid-conjugated carbon dots have been used for sensitive analysis of glucose with high selectivity towards interferent species (Pan et al., 2018).
Covalent Organic Frameworks
- Research Focus : Synthesis of covalent organic frameworks (COFs) using 1,3-benzenediboronic acid as a precursor. This includes the development of distinct polymorphs and the creation of Sierpiński triangles by on-surface synthesis (Cui et al., 2019).
Supramolecular Chemistry
- Research Focus : Multicomponent assembly of macrocycles and polymers through coordination of pyridyl ligands to 1,4-bis(benzodioxaborole)benzene, demonstrating the utility of dative B-N bonds in structural supramolecular chemistry and crystal engineering (Içli et al., 2012).
Crystal Engineering
- Research Focus : Investigating the structural and reactivity properties of cocrystals involving diboronic acids and bipyridines, focusing on hydrogen-bonded architectures and photodimerization processes (Vasquez-Ríos et al., 2022).
Polymer Synthesis
- Research Focus : Synthesis of rigid rod poly(p-phenylenes) using this compound derivatives, exploring the solubility and molecular properties of these polymers (Vanhee et al., 1996).
Electrochemical Studies
- Research Focus : Electrochemical oxidation studies of caffeic acid in the presence of 1,4-benzenediboronic acid, exploring complex formation mechanisms and glucose effects on these complexes (Beiginejad et al., 2019).
Safety and Hazards
Orientations Futures
Mécanisme D'action
Target of Action
1,3-Benzenediboronic acid is a chemical compound with the molecular formula C6H8B2O4
Biochemical Pathways
It’s worth noting that boronic acids are often used in the synthesis of various pharmaceuticals and bioactive compounds, suggesting they may interact with a wide range of biochemical pathways .
Pharmacokinetics
It’s known that the compound is slightly soluble in water , which may influence its bioavailability.
Result of Action
One study mentions that this compound is used to prepare 1,3-dihydroxybenzene by oxidation reaction using alkaline hydrogen peroxide .
Action Environment
It’s known that the compound should be stored under inert gas (nitrogen or argon) at 2-8°c , suggesting that its stability may be affected by exposure to oxygen and variations in temperature.
Analyse Biochimique
Biochemical Properties
1,3-Benzenediboronic acid plays a significant role in biochemical reactions, particularly in the formation of cocrystals and supramolecular assemblies. It interacts with various enzymes, proteins, and biomolecules through hydrogen bonding and other non-covalent interactions. For instance, it forms hydrogen-bonded cocrystals with bipyridines, which are sustained by interactions such as B(O)H···N, B(O)H···O, and π···π interactions . These interactions facilitate the formation of stable and reactive structures, making this compound a valuable building block in supramolecular chemistry.
Cellular Effects
This compound influences various cellular processes and functions. It has been observed to affect cell signaling pathways, gene expression, and cellular metabolism. The compound’s ability to form stable cocrystals and interact with biomolecules can lead to changes in cellular behavior and function. For example, its interactions with proteins and enzymes can modulate their activity, leading to alterations in metabolic pathways and gene expression . These effects highlight the potential of this compound in regulating cellular processes and functions.
Molecular Mechanism
The molecular mechanism of this compound involves its ability to form hydrogen bonds and other non-covalent interactions with biomolecules. These interactions can lead to enzyme inhibition or activation, changes in gene expression, and modulation of cellular signaling pathways. For instance, the compound’s interaction with bipyridines results in the formation of hydrogen-bonded cocrystals, which can undergo photodimerization upon UV radiation . This photodimerization process highlights the compound’s potential in modulating molecular interactions and biochemical reactions.
Temporal Effects in Laboratory Settings
In laboratory settings, the effects of this compound can change over time due to its stability and degradation properties. The compound’s stability in various conditions, including its resistance to degradation, plays a crucial role in its long-term effects on cellular function. Studies have shown that this compound can form stable cocrystals that maintain their structure and reactivity over time . These properties make it a valuable tool for long-term biochemical studies and applications.
Dosage Effects in Animal Models
The effects of this compound in animal models vary with different dosages. At lower doses, the compound may exhibit beneficial effects on cellular processes and functions, while higher doses may lead to toxic or adverse effects. Studies have shown that the compound’s acute oral toxicity is categorized as harmful if swallowed . Therefore, careful consideration of dosage is essential when using this compound in animal studies to avoid potential toxic effects.
Metabolic Pathways
This compound is involved in various metabolic pathways, interacting with enzymes and cofactors that regulate metabolic flux and metabolite levels. The compound’s ability to form stable interactions with biomolecules can influence metabolic processes, such as the tricarboxylic acid cycle and oxidative phosphorylation . These interactions highlight the compound’s potential in modulating metabolic pathways and influencing cellular metabolism.
Transport and Distribution
The transport and distribution of this compound within cells and tissues involve interactions with transporters and binding proteins. These interactions can affect the compound’s localization and accumulation within specific cellular compartments. For instance, the compound’s ability to form stable cocrystals can influence its distribution and transport within cells . Understanding these interactions is crucial for optimizing the compound’s use in biochemical applications.
Subcellular Localization
The subcellular localization of this compound is influenced by its interactions with targeting signals and post-translational modifications. These interactions can direct the compound to specific compartments or organelles within the cell, affecting its activity and function. For example, the compound’s ability to form hydrogen-bonded cocrystals can influence its localization within the cell . These properties highlight the importance of understanding the compound’s subcellular localization for its effective use in biochemical research.
Propriétés
IUPAC Name |
(3-boronophenyl)boronic acid | |
---|---|---|
Source | PubChem | |
URL | https://pubchem.ncbi.nlm.nih.gov | |
Description | Data deposited in or computed by PubChem | |
InChI |
InChI=1S/C6H8B2O4/c9-7(10)5-2-1-3-6(4-5)8(11)12/h1-4,9-12H | |
Source | PubChem | |
URL | https://pubchem.ncbi.nlm.nih.gov | |
Description | Data deposited in or computed by PubChem | |
InChI Key |
UXPAASVRXBULRG-UHFFFAOYSA-N | |
Source | PubChem | |
URL | https://pubchem.ncbi.nlm.nih.gov | |
Description | Data deposited in or computed by PubChem | |
Canonical SMILES |
B(C1=CC(=CC=C1)B(O)O)(O)O | |
Source | PubChem | |
URL | https://pubchem.ncbi.nlm.nih.gov | |
Description | Data deposited in or computed by PubChem | |
Molecular Formula |
C6H8B2O4 | |
Source | PubChem | |
URL | https://pubchem.ncbi.nlm.nih.gov | |
Description | Data deposited in or computed by PubChem | |
DSSTOX Substance ID |
DTXSID10282315 | |
Record name | 1,3-benzenediboronic acid | |
Source | EPA DSSTox | |
URL | https://comptox.epa.gov/dashboard/DTXSID10282315 | |
Description | DSSTox provides a high quality public chemistry resource for supporting improved predictive toxicology. | |
Molecular Weight |
165.75 g/mol | |
Source | PubChem | |
URL | https://pubchem.ncbi.nlm.nih.gov | |
Description | Data deposited in or computed by PubChem | |
CAS No. |
4612-28-6 | |
Record name | 4612-28-6 | |
Source | DTP/NCI | |
URL | https://dtp.cancer.gov/dtpstandard/servlet/dwindex?searchtype=NSC&outputformat=html&searchlist=25409 | |
Description | The NCI Development Therapeutics Program (DTP) provides services and resources to the academic and private-sector research communities worldwide to facilitate the discovery and development of new cancer therapeutic agents. | |
Explanation | Unless otherwise indicated, all text within NCI products is free of copyright and may be reused without our permission. Credit the National Cancer Institute as the source. | |
Record name | 1,3-benzenediboronic acid | |
Source | EPA DSSTox | |
URL | https://comptox.epa.gov/dashboard/DTXSID10282315 | |
Description | DSSTox provides a high quality public chemistry resource for supporting improved predictive toxicology. | |
Record name | 1,3-Benzenediboronic acid | |
Source | European Chemicals Agency (ECHA) | |
URL | https://echa.europa.eu/information-on-chemicals | |
Description | The European Chemicals Agency (ECHA) is an agency of the European Union which is the driving force among regulatory authorities in implementing the EU's groundbreaking chemicals legislation for the benefit of human health and the environment as well as for innovation and competitiveness. | |
Explanation | Use of the information, documents and data from the ECHA website is subject to the terms and conditions of this Legal Notice, and subject to other binding limitations provided for under applicable law, the information, documents and data made available on the ECHA website may be reproduced, distributed and/or used, totally or in part, for non-commercial purposes provided that ECHA is acknowledged as the source: "Source: European Chemicals Agency, http://echa.europa.eu/". Such acknowledgement must be included in each copy of the material. ECHA permits and encourages organisations and individuals to create links to the ECHA website under the following cumulative conditions: Links can only be made to webpages that provide a link to the Legal Notice page. | |
Retrosynthesis Analysis
AI-Powered Synthesis Planning: Our tool employs the Template_relevance Pistachio, Template_relevance Bkms_metabolic, Template_relevance Pistachio_ringbreaker, Template_relevance Reaxys, Template_relevance Reaxys_biocatalysis model, leveraging a vast database of chemical reactions to predict feasible synthetic routes.
One-Step Synthesis Focus: Specifically designed for one-step synthesis, it provides concise and direct routes for your target compounds, streamlining the synthesis process.
Accurate Predictions: Utilizing the extensive PISTACHIO, BKMS_METABOLIC, PISTACHIO_RINGBREAKER, REAXYS, REAXYS_BIOCATALYSIS database, our tool offers high-accuracy predictions, reflecting the latest in chemical research and data.
Strategy Settings
Precursor scoring | Relevance Heuristic |
---|---|
Min. plausibility | 0.01 |
Model | Template_relevance |
Template Set | Pistachio/Bkms_metabolic/Pistachio_ringbreaker/Reaxys/Reaxys_biocatalysis |
Top-N result to add to graph | 6 |
Feasible Synthetic Routes
Q1: How does 1,3-Benzenediboronic acid interact with other molecules, and what are the downstream effects of these interactions?
A: this compound (1,3-BDBA) primarily interacts with molecules containing diols or polyols through the formation of boronate ester bonds. This interaction is reversible and sensitive to pH and the presence of oxidizing agents like hydrogen peroxide. []
- On-surface synthesis: 1,3-BDBA can undergo dehydration reactions with water on surfaces, leading to the formation of covalent organic frameworks, such as Sierpiński triangles. [, ] This self-assembly process is influenced by the concentration of 1,3-BDBA. []
- Polymer synthesis: 1,3-BDBA reacts with diols or polyols to form polymers. For example, it reacts with 5-octyloxy-1,3-benzenediboronic acid pinacolate to form all-cis poly(m-phenylenevinylene)s (PmPVs) via Suzuki-Miyaura polycondensation. [] It can also form mesoporous polyboronate materials with hexane-1,2,5,6-tetrol, with properties influenced by the diastereomeric excess of the tetrol and the orientation of the boronic acid groups. []
- Stimuli-responsive materials: The boronate ester bonds formed by 1,3-BDBA are susceptible to oxidation by hydrogen peroxide. This property allows the creation of stimuli-responsive hydrogels where the release of encapsulated molecules, like insulin, can be triggered by H2O2. []
Q2: What is the structural characterization of this compound?
A2: this compound consists of a benzene ring with two boronic acid (-B(OH)2) groups substituted at the 1 and 3 positions.
Q3: Can you explain the material compatibility and stability of this compound in various applications?
A3: 1,3-BDBA demonstrates versatility in material science due to its ability to form robust covalent bonds and self-assemble into ordered structures.
- Covalent Organic Frameworks (COFs): 1,3-BDBA serves as a reliable building block for COFs. Its use allows for the creation of materials with varying pore sizes and crystallinity, depending on reaction conditions. [, ]
- Stimuli-Responsive Materials: The sensitivity of boronate ester bonds to oxidation by H2O2 makes 1,3-BDBA suitable for developing materials with controlled release properties. This has potential applications in drug delivery systems. []
- Polymers: 1,3-BDBA enables the synthesis of polymers like PmPVs, with potential applications in organic electronics and photonics. []
Q4: What are the cocrystallization properties of this compound?
A: 1,3-BDBA forms cocrystals with bipyridines like 1,2-bis(4-pyridyl)ethylene (bpe) and 1,2-bis(4-pyridyl)ethane (bpeta) through hydrogen bonding interactions. [] These interactions involve the boronic acid groups of 1,3-BDBA and the nitrogen atoms of the bipyridine molecules. These cocrystals can exhibit interesting reactivities, such as photodimerization of bpe upon UV irradiation, leading to the formation of cyclobutane. [] This highlights the potential of using 1,3-BDBA in crystal engineering and designing materials with photo-responsive properties.
Avertissement et informations sur les produits de recherche in vitro
Veuillez noter que tous les articles et informations sur les produits présentés sur BenchChem sont destinés uniquement à des fins informatives. Les produits disponibles à l'achat sur BenchChem sont spécifiquement conçus pour des études in vitro, qui sont réalisées en dehors des organismes vivants. Les études in vitro, dérivées du terme latin "in verre", impliquent des expériences réalisées dans des environnements de laboratoire contrôlés à l'aide de cellules ou de tissus. Il est important de noter que ces produits ne sont pas classés comme médicaments et n'ont pas reçu l'approbation de la FDA pour la prévention, le traitement ou la guérison de toute condition médicale, affection ou maladie. Nous devons souligner que toute forme d'introduction corporelle de ces produits chez les humains ou les animaux est strictement interdite par la loi. Il est essentiel de respecter ces directives pour assurer la conformité aux normes légales et éthiques en matière de recherche et d'expérimentation.