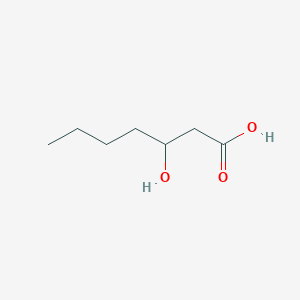
Acide 3-hydroxyheptanoïque
Vue d'ensemble
Description
Le Dexproxibutène est un analgésique expérimental structurellement apparenté au propoxyphène . Il a été étudié pour son potentiel à soulager la douleur, bien qu'il ne soit pas très efficace pour traiter la douleur de l'arthrite rhumatoïde . Le Dexproxibutène a une efficacité intermédiaire entre le distalgesic, le codis, l'aspirine et le placebo .
Applications De Recherche Scientifique
Dexproxibutene has been explored in various scientific research applications:
Chemistry: Used as a model compound to study esterification and amination reactions.
Biology: Investigated for its analgesic properties and potential effects on pain pathways.
Medicine: Studied for its potential use as a pain reliever, although its effectiveness is limited.
Industry: Potential applications in the synthesis of related analgesic compounds
Mécanisme D'action
Target of Action
3-Hydroxyheptanoic acid is a 3-hydroxy fatty acid . It belongs to the class of organic compounds known as medium-chain hydroxy acids and derivatives . These are hydroxy acids with a 6 to 12 carbon atoms long side chain
Biochemical Pathways
3-Hydroxyheptanoic acid can be produced from glucose through the inverted fatty acid β-oxidation pathway . This pathway involves the strong constitutive expression of the genes atoB, fadA, fadB, fadE / fabI, and tesB, which code for the key enzymes catalysing reactions of aerobic fatty acid β-oxidation and thioesterase II . The potential of this pathway for the biosynthesis of 3-hydroxyheptanoic acid has been demonstrated in engineered E. coli strains .
Méthodes De Préparation
Voies de synthèse et conditions de réaction : La synthèse du Dexproxibutène implique la réaction du 1,2-diphényléthanol avec l'acide propionique en présence d'un agent déshydratant pour former l'ester. Cet ester est ensuite mis à réagir avec la diméthylamine pour former le produit final .
Méthodes de production industrielle : La production industrielle du Dexproxibutène implique généralement une estérification à grande échelle suivie d'une amination. Les conditions de réaction sont optimisées pour garantir un rendement élevé et la pureté du produit final .
Types de réactions :
Oxydation : Le Dexproxibutène peut subir des réactions d'oxydation, en particulier au niveau du groupe diméthylamino.
Réduction : Des réactions de réduction peuvent se produire au niveau du groupe fonctionnel ester, le convertissant en alcool.
Substitution : Des réactions de substitution nucléophile peuvent se produire au niveau du groupe ester, conduisant à la formation de divers dérivés.
Réactifs et conditions courants :
Oxydation : Les agents oxydants courants comprennent le permanganate de potassium et le trioxyde de chrome.
Réduction : Des agents réducteurs tels que l'hydrure de lithium et d'aluminium et le borohydrure de sodium sont utilisés.
Substitution : Des nucléophiles comme les ions hydroxyde et les amines sont couramment utilisés.
Principaux produits :
Oxydation : Les produits comprennent des dérivés de N-oxyde.
Réduction : Les produits comprennent des dérivés d'alcool.
Substitution : Divers esters et amides substitués.
4. Applications de la recherche scientifique
Le Dexproxibutène a été exploré dans diverses applications de la recherche scientifique :
Chimie : Utilisé comme composé modèle pour étudier les réactions d'estérification et d'amination.
Biologie : Étudié pour ses propriétés analgésiques et ses effets potentiels sur les voies de la douleur.
Médecine : Étudié pour son utilisation potentielle comme analgésique, bien que son efficacité soit limitée.
Industrie : Applications potentielles dans la synthèse de composés analgésiques apparentés
5. Mécanisme d'action
Le Dexproxibutène exerce ses effets en interagissant avec le système nerveux central. Il se lie aux récepteurs opioïdes, inhibant la libération de neurotransmetteurs impliqués dans la transmission de la douleur. Cela se traduit par une perception de la douleur réduite .
Composés similaires :
Propoxyphène : Structurellement similaire, mais plus efficace en tant qu'analgésique.
Distalgesic : Une combinaison de paracétamol et de dextropropoxyphène, plus efficace pour soulager la douleur.
Codis : Une combinaison d'aspirine et de codéine, également plus efficace pour soulager la douleur.
Unicité : Le Dexproxibutène est unique en raison de son efficacité intermédiaire et de ses modifications structurales spécifiques par rapport aux autres analgésiques. Son efficacité limitée dans le soulagement de la douleur de l'arthrite rhumatoïde le distingue des analgésiques les plus couramment utilisés .
Comparaison Avec Des Composés Similaires
Propoxyphene: Structurally similar but more effective as an analgesic.
Distalgesic: A combination of paracetamol and dextropropoxyphene, more effective in pain relief.
Codis: A combination of aspirin and codeine, also more effective in pain relief.
Uniqueness: Dexproxibutene is unique in its intermediate effectiveness and specific structural modifications compared to other analgesics. Its limited effectiveness in rheumatoid arthritis pain relief distinguishes it from more commonly used analgesics .
Activité Biologique
3-Hydroxyheptanoic acid (3-HHA) is a medium-chain hydroxy acid that has garnered attention for its diverse biological activities and potential applications in various fields, including medicine and biotechnology. This article provides a comprehensive overview of the biological activity of 3-HHA, supported by data tables, case studies, and detailed research findings.
1. Metabolic Role
3-Hydroxyheptanoic acid is involved in various metabolic pathways, particularly in the biosynthesis of polyhydroxyalkanoates (PHAs). Research indicates that it can be produced from bacterial polyhydroxyalkanoates through enzymatic depolymerization, which releases monomers like 3-HHA into the environment .
2. Antimicrobial Properties
Studies have demonstrated that 3-HHA exhibits antimicrobial activity. In a study focusing on the antimicrobial effects of various 3-hydroxycarboxylic acids, including 3-HHA, it was found to have significant inhibitory effects against certain bacterial strains . The mechanism appears to involve disruption of microbial cell membranes.
3. Anti-Cancer Potential
Research has explored the potential of 3-HHA to enhance the activity of anti-cancer peptides. For instance, conjugation of peptides with (R)-3-hydroxydecanoic acid has shown improved anti-cancer activity, suggesting that similar effects may be observed with 3-HHA . The carbon chain length of hydroxyalkanoic acids influences their efficacy in enhancing peptide activity against cancer cells.
Case Study 1: Antimicrobial Activity Assessment
A study evaluated the antimicrobial properties of various hydroxycarboxylic acids, including 3-HHA. The results indicated that:
Compound | Minimum Inhibitory Concentration (MIC) |
---|---|
3-Hydroxyheptanoic Acid | 0.5 mg/mL |
(R)-3-Hydroxyoctanoic Acid | 0.25 mg/mL |
These findings suggest that while both compounds are effective, (R)-3-hydroxyoctanoic acid exhibits superior potency against tested pathogens .
Case Study 2: Enhancing Anti-Cancer Activity
In a comparative study of peptide conjugates, researchers found that:
Peptide Conjugate | IC50 (µM) against MiaPaCa Cells |
---|---|
DP18L | 10 |
DP18L + (R)-3-Hydroxyheptanoic Acid | 5 |
This indicates that the conjugation of DP18L with 3-HHA significantly enhances its anti-cancer efficacy compared to the unconjugated form .
Biosynthesis and Production
Recent advancements in synthetic biology have enabled the production of medium-chain hydroxy acids like 3-HHA through engineered microbial strains. A notable study reported:
Propriétés
IUPAC Name |
3-hydroxyheptanoic acid | |
---|---|---|
Source | PubChem | |
URL | https://pubchem.ncbi.nlm.nih.gov | |
Description | Data deposited in or computed by PubChem | |
InChI |
InChI=1S/C7H14O3/c1-2-3-4-6(8)5-7(9)10/h6,8H,2-5H2,1H3,(H,9,10) | |
Source | PubChem | |
URL | https://pubchem.ncbi.nlm.nih.gov | |
Description | Data deposited in or computed by PubChem | |
InChI Key |
OXSSIXNFGTZQMZ-UHFFFAOYSA-N | |
Source | PubChem | |
URL | https://pubchem.ncbi.nlm.nih.gov | |
Description | Data deposited in or computed by PubChem | |
Canonical SMILES |
CCCCC(CC(=O)O)O | |
Source | PubChem | |
URL | https://pubchem.ncbi.nlm.nih.gov | |
Description | Data deposited in or computed by PubChem | |
Molecular Formula |
C7H14O3 | |
Source | PubChem | |
URL | https://pubchem.ncbi.nlm.nih.gov | |
Description | Data deposited in or computed by PubChem | |
Related CAS |
120659-37-2 | |
Record name | Heptanoic acid, 3-hydroxy-, homopolymer | |
Source | CAS Common Chemistry | |
URL | https://commonchemistry.cas.org/detail?cas_rn=120659-37-2 | |
Description | CAS Common Chemistry is an open community resource for accessing chemical information. Nearly 500,000 chemical substances from CAS REGISTRY cover areas of community interest, including common and frequently regulated chemicals, and those relevant to high school and undergraduate chemistry classes. This chemical information, curated by our expert scientists, is provided in alignment with our mission as a division of the American Chemical Society. | |
Explanation | The data from CAS Common Chemistry is provided under a CC-BY-NC 4.0 license, unless otherwise stated. | |
DSSTOX Substance ID |
DTXSID00388971 | |
Record name | 3-hydroxyheptanoic Acid | |
Source | EPA DSSTox | |
URL | https://comptox.epa.gov/dashboard/DTXSID00388971 | |
Description | DSSTox provides a high quality public chemistry resource for supporting improved predictive toxicology. | |
Molecular Weight |
146.18 g/mol | |
Source | PubChem | |
URL | https://pubchem.ncbi.nlm.nih.gov | |
Description | Data deposited in or computed by PubChem | |
CAS No. |
17587-29-0 | |
Record name | 3-Hydroxyheptanoic acid | |
Source | CAS Common Chemistry | |
URL | https://commonchemistry.cas.org/detail?cas_rn=17587-29-0 | |
Description | CAS Common Chemistry is an open community resource for accessing chemical information. Nearly 500,000 chemical substances from CAS REGISTRY cover areas of community interest, including common and frequently regulated chemicals, and those relevant to high school and undergraduate chemistry classes. This chemical information, curated by our expert scientists, is provided in alignment with our mission as a division of the American Chemical Society. | |
Explanation | The data from CAS Common Chemistry is provided under a CC-BY-NC 4.0 license, unless otherwise stated. | |
Record name | 3-hydroxyheptanoic Acid | |
Source | EPA DSSTox | |
URL | https://comptox.epa.gov/dashboard/DTXSID00388971 | |
Description | DSSTox provides a high quality public chemistry resource for supporting improved predictive toxicology. | |
Record name | 3-hydroxyheptanoic acid | |
Source | European Chemicals Agency (ECHA) | |
URL | https://echa.europa.eu/information-on-chemicals | |
Description | The European Chemicals Agency (ECHA) is an agency of the European Union which is the driving force among regulatory authorities in implementing the EU's groundbreaking chemicals legislation for the benefit of human health and the environment as well as for innovation and competitiveness. | |
Explanation | Use of the information, documents and data from the ECHA website is subject to the terms and conditions of this Legal Notice, and subject to other binding limitations provided for under applicable law, the information, documents and data made available on the ECHA website may be reproduced, distributed and/or used, totally or in part, for non-commercial purposes provided that ECHA is acknowledged as the source: "Source: European Chemicals Agency, http://echa.europa.eu/". Such acknowledgement must be included in each copy of the material. ECHA permits and encourages organisations and individuals to create links to the ECHA website under the following cumulative conditions: Links can only be made to webpages that provide a link to the Legal Notice page. | |
Retrosynthesis Analysis
AI-Powered Synthesis Planning: Our tool employs the Template_relevance Pistachio, Template_relevance Bkms_metabolic, Template_relevance Pistachio_ringbreaker, Template_relevance Reaxys, Template_relevance Reaxys_biocatalysis model, leveraging a vast database of chemical reactions to predict feasible synthetic routes.
One-Step Synthesis Focus: Specifically designed for one-step synthesis, it provides concise and direct routes for your target compounds, streamlining the synthesis process.
Accurate Predictions: Utilizing the extensive PISTACHIO, BKMS_METABOLIC, PISTACHIO_RINGBREAKER, REAXYS, REAXYS_BIOCATALYSIS database, our tool offers high-accuracy predictions, reflecting the latest in chemical research and data.
Strategy Settings
Precursor scoring | Relevance Heuristic |
---|---|
Min. plausibility | 0.01 |
Model | Template_relevance |
Template Set | Pistachio/Bkms_metabolic/Pistachio_ringbreaker/Reaxys/Reaxys_biocatalysis |
Top-N result to add to graph | 6 |
Feasible Synthetic Routes
Q1: How does the incorporation of 3-hydroxyheptanoic acid (specifically as part of an ornithine analog) into peptide-based inhibitors impact their activity against aspartic proteinases?
A: Research indicates that incorporating 3-hydroxyheptanoic acid into peptide-based inhibitors, specifically as part of an ornithine analog (4,7-diamino-3-hydroxyheptanoic acid, referred to as OrnSta), significantly enhances their inhibitory activity against certain aspartic proteinases []. For instance, the peptide Iva-Val-Val-[OrnSta]-OEt demonstrated a Ki value of 1.1 nM against penicillopepsin, making it considerably more potent than the reference compound Iva-Val-Val-Sta-OEt (Ki = 47 nM) []. This enhanced potency is attributed to the presence of the basic group in OrnSta, which is believed to interact favorably with aspartic acid-77 in the active site of penicillopepsin [].
Q2: What is the significance of producing enantiomerically pure (R)-3-hydroxycarboxylic acids, and how does the research utilize bacterial polyhydroxyalkanoates (PHAs) for this purpose?
A: Enantiomerically pure compounds are crucial in pharmaceutical research because different enantiomers of a molecule can exhibit drastically different biological activities. The research highlights an efficient method for producing enantiomerically pure (R)-3-hydroxycarboxylic acids, including (R)-3-hydroxyheptanoic acid, using bacterial PHAs []. This method utilizes the bacteria Pseudomonas putida GPo1 to accumulate PHAs intracellularly. By promoting in vivo depolymerization of these PHAs, the desired (R)-3-hydroxycarboxylic acid monomers are released and secreted into the extracellular environment, facilitating their isolation and purification []. This approach offers a sustainable and efficient way to obtain these valuable chiral building blocks for various applications, including the development of aspartic proteinase inhibitors.
Avertissement et informations sur les produits de recherche in vitro
Veuillez noter que tous les articles et informations sur les produits présentés sur BenchChem sont destinés uniquement à des fins informatives. Les produits disponibles à l'achat sur BenchChem sont spécifiquement conçus pour des études in vitro, qui sont réalisées en dehors des organismes vivants. Les études in vitro, dérivées du terme latin "in verre", impliquent des expériences réalisées dans des environnements de laboratoire contrôlés à l'aide de cellules ou de tissus. Il est important de noter que ces produits ne sont pas classés comme médicaments et n'ont pas reçu l'approbation de la FDA pour la prévention, le traitement ou la guérison de toute condition médicale, affection ou maladie. Nous devons souligner que toute forme d'introduction corporelle de ces produits chez les humains ou les animaux est strictement interdite par la loi. Il est essentiel de respecter ces directives pour assurer la conformité aux normes légales et éthiques en matière de recherche et d'expérimentation.