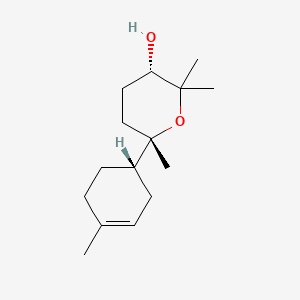
Bisabolol oxide A
Vue d'ensemble
Description
Bisabolol oxide A is a naturally occurring sesquiterpene alcohol derived from the essential oils of various plants, notably German chamomile (Matricaria recutita). It is a colorless, viscous oil known for its anti-inflammatory, antimicrobial, and skin-soothing properties. This compound is a significant constituent of chamomile oil and has been used in traditional medicine for its therapeutic benefits .
Mécanisme D'action
Target of Action
Bisabolol oxide A, a constituent of German chamomile, has been found to regulate T-cell lymphatic subpopulations, inhibiting the Th17 cell differentiation signaling pathway . This results in a reduction of interleukin 17 (IL-17), thereby inhibiting the activation of the nuclear factor kappa beta (NF-κB) and MAPK pathways . It also inhibits cathepsin D and ornithine decarboxylase .
Mode of Action
This compound interacts with its targets, leading to a decrease in the secretion of pro-inflammatory factors such as tumor necrosis factor alpha (TNF-α) and interleukin 6 (IL-6), thus reducing inflammation . It also influences the action of inflammatory mediators involved in the second phase of the formalin test .
Biochemical Pathways
The biosynthesis pathway of α-bisabolol, to which this compound belongs, is part of the sesquiterpenoids pathway. The MEP and MVA pathways acting upstream provide IPP, and farnesyl diphosphate synthase (FPS) forms the important intermediate product (FPP) by catalyzing the reaction of DMAPP and two molecules of IPP .
Result of Action
The molecular and cellular effects of this compound’s action include a reduction in the secretion of pro-inflammatory factors (TNF-α and IL-6), leading to a decrease in inflammation . It also has anti-nociceptive activity, influencing the action of inflammatory mediators .
Action Environment
This compound is currently manufactured mainly by steam-distillation of the essential oils extracted from the Brazilian candeia tree . Microbial production of this compound plays a key role in the development of its sustainable production from renewable feedstock . The engineered E. coli strains and economically viable extraction process developed in this study will serve as promising platforms for further development of microbial production of this compound at large scale .
Analyse Biochimique
Biochemical Properties
Bisabolol oxide A plays a crucial role in various biochemical reactions. It interacts with several enzymes, proteins, and other biomolecules, influencing their activity and function. One of the key interactions of this compound is with the enzyme farnesyl diphosphate synthase, which is involved in the biosynthesis of sesquiterpenoids. This interaction facilitates the production of this compound from its precursor, farnesyl diphosphate . Additionally, this compound has been shown to interact with various proteins involved in inflammatory pathways, such as cyclooxygenase-2 (COX-2) and inducible nitric oxide synthase (iNOS), inhibiting their activity and thereby exerting anti-inflammatory effects .
Cellular Effects
This compound exerts significant effects on various types of cells and cellular processes. It has been demonstrated to influence cell function by modulating cell signaling pathways, gene expression, and cellular metabolism. For instance, this compound has been shown to inhibit the activation of nuclear factor-kappa B (NF-κB), a key transcription factor involved in inflammatory responses . This inhibition leads to a decrease in the expression of pro-inflammatory cytokines such as tumor necrosis factor-alpha (TNF-α) and interleukin-6 (IL-6) . Furthermore, this compound has been reported to induce apoptosis in cancer cells by upregulating the expression of pro-apoptotic genes and downregulating anti-apoptotic genes .
Molecular Mechanism
The molecular mechanism of action of this compound involves several key processes. At the molecular level, this compound exerts its effects through binding interactions with biomolecules, enzyme inhibition, and changes in gene expression. One of the primary mechanisms is the inhibition of COX-2 and iNOS enzymes, which are involved in the production of pro-inflammatory mediators . By inhibiting these enzymes, this compound reduces the levels of inflammatory mediators, thereby exerting anti-inflammatory effects. Additionally, this compound has been shown to modulate the expression of genes involved in apoptosis, leading to the induction of programmed cell death in cancer cells .
Temporal Effects in Laboratory Settings
In laboratory settings, the effects of this compound have been observed to change over time. Studies have shown that this compound is relatively stable under standard laboratory conditions, with minimal degradation over extended periods . Its long-term effects on cellular function have been investigated in both in vitro and in vivo studies. In vitro studies have demonstrated that prolonged exposure to this compound can lead to sustained anti-inflammatory and anticancer effects . In vivo studies have also shown that this compound maintains its pharmacological activity over time, with no significant loss of efficacy .
Dosage Effects in Animal Models
The effects of this compound vary with different dosages in animal models. Studies have shown that low to moderate doses of this compound exhibit significant therapeutic effects, including anti-inflammatory, analgesic, and anticancer activities . At higher doses, this compound may exhibit toxic or adverse effects. For instance, high doses of this compound have been associated with hepatotoxicity and nephrotoxicity in animal models . Therefore, it is crucial to determine the optimal dosage range to maximize the therapeutic benefits while minimizing potential adverse effects.
Metabolic Pathways
This compound is involved in several metabolic pathways, including the sesquiterpenoid biosynthesis pathway. The biosynthesis of this compound involves the conversion of farnesyl diphosphate to this compound through the action of farnesyl diphosphate synthase and Bisabolol oxide synthase . Additionally, this compound interacts with various enzymes and cofactors involved in its metabolism, influencing metabolic flux and metabolite levels . These interactions play a crucial role in determining the pharmacokinetics and pharmacodynamics of this compound.
Transport and Distribution
The transport and distribution of this compound within cells and tissues are mediated by specific transporters and binding proteins. Studies have shown that this compound is transported across cell membranes through passive diffusion and active transport mechanisms . Once inside the cells, this compound can bind to intracellular proteins, influencing its localization and accumulation. The distribution of this compound within tissues is also influenced by its lipophilic nature, allowing it to accumulate in lipid-rich tissues .
Subcellular Localization
The subcellular localization of this compound plays a crucial role in its activity and function. Studies have shown that this compound is primarily localized in the cytosol, where it interacts with various enzymes and proteins involved in its biochemical and pharmacological effects . Additionally, this compound may undergo post-translational modifications that direct it to specific subcellular compartments or organelles, further influencing its activity and function .
Méthodes De Préparation
Synthetic Routes and Reaction Conditions: Bisabolol oxide A can be synthesized through the microbial transformation of α-bisabolol. This process involves the use of fungi such as Thamnidium elegans and Penicillium neocrassum, which convert α-bisabolol to this compound under controlled conditions . The reaction typically occurs at ambient temperature and neutral pH, with the fungi acting as biocatalysts.
Industrial Production Methods: Industrial production of this compound often involves the extraction of α-bisabolol from natural sources like chamomile oil, followed by its oxidation. The oxidation process can be carried out using oxidizing agents such as hydrogen peroxide or ozone under mild conditions to yield this compound .
Analyse Des Réactions Chimiques
Types of Reactions: Bisabolol oxide A undergoes various chemical reactions, including:
Oxidation: Conversion of α-bisabolol to this compound using oxidizing agents.
Reduction: Reduction of this compound to its corresponding alcohol.
Substitution: Substitution reactions involving the hydroxyl group of this compound.
Common Reagents and Conditions:
Oxidizing Agents: Hydrogen peroxide, ozone.
Reducing Agents: Sodium borohydride, lithium aluminum hydride.
Solvents: Ethanol, methanol, water.
Major Products:
Oxidation: this compound.
Reduction: α-bisabolol.
Substitution: Various substituted bisabolol derivatives
Applications De Recherche Scientifique
Bisabolol oxide A has a wide range of applications in scientific research:
Chemistry: Used as a precursor for synthesizing other bioactive compounds.
Biology: Studied for its antimicrobial and anti-inflammatory properties.
Medicine: Investigated for its potential in treating skin conditions, inflammation, and microbial infections.
Industry: Utilized in cosmetics and personal care products for its skin-soothing and healing properties.
Comparaison Avec Des Composés Similaires
α-Bisabolol: The parent compound, known for its anti-inflammatory and antimicrobial properties.
Bisabolol oxide B: Another oxidation product of α-bisabolol with similar properties.
Chamomile oil constituents: Other sesquiterpenes found in chamomile oil, such as chamazulene and farnesene.
Uniqueness: Bisabolol oxide A is unique due to its specific oxidation state and structural configuration, which confer distinct biological activities. Its enhanced solubility and stability compared to α-bisabolol make it a valuable compound in various applications .
Activité Biologique
Bisabolol oxide A, a derivative of α-bisabolol, is a naturally occurring compound found in various essential oils, particularly from the Matricaria chamomilla (chamomile) plant. This compound has garnered attention due to its diverse biological activities, including anti-inflammatory, antioxidant, and neuroprotective effects. This article reviews the current understanding of the biological activity of this compound, supported by recent research findings and case studies.
This compound is characterized by its sesquiterpene structure, which contributes to its pharmacological properties. It is often present in essential oils alongside other compounds like α-bisabolol and β-farnesene. Its unique structure allows it to interact with various biological pathways, making it a subject of interest in pharmacology.
1. Antioxidant Activity
Research indicates that this compound exhibits significant antioxidant properties. It has been shown to scavenge free radicals effectively, with an IC50 value of 1.50 mg/mL for DPPH radical scavenging ability, outperforming α-bisabolol (IC50 of 43.88 mg/mL) . This antioxidant capacity is crucial in mitigating oxidative stress-related damage in cells.
2. Anti-inflammatory Effects
This compound has demonstrated potent anti-inflammatory effects in various models. In a study involving a rat model of inflammation induced by carrageenan, bisabolol oxide-rich matricaria oil significantly reduced paw edema and hyperalgesia . The mechanism involves the inhibition of pro-inflammatory cytokines such as IL-1β and TNF-α, along with a reduction in the expression of COX-2 and iNOS .
3. Neuroprotective Properties
The neuroprotective potential of this compound has been highlighted in studies focusing on neurodegenerative diseases such as Parkinson's and Alzheimer's disease. In models of Parkinson's disease induced by rotenone, this compound treatment resulted in the preservation of dopaminergic neurons and reduced oxidative stress markers like malondialdehyde (MDA) . Additionally, it showed promise in protecting against amyloid-beta-induced neurotoxicity by reducing reactive oxygen species (ROS) levels and enhancing cellular viability .
Research Findings and Case Studies
The biological activities of this compound can be attributed to several mechanisms:
- Antioxidant Mechanism : this compound enhances the expression of antioxidant proteins such as superoxide dismutase (SOD) and catalase (CAT), effectively reducing oxidative damage in cells.
- Anti-inflammatory Mechanism : It inhibits the activation of glial cells and reduces the release of pro-inflammatory cytokines, thereby alleviating inflammation.
- Neuroprotective Mechanism : By restoring mitochondrial function and preventing lipid peroxidation, this compound protects neuronal integrity against toxic insults.
Propriétés
IUPAC Name |
2,2,6-trimethyl-6-(4-methylcyclohex-3-en-1-yl)oxan-3-ol | |
---|---|---|
Details | Computed by Lexichem TK 2.7.0 (PubChem release 2021.05.07) | |
Source | PubChem | |
URL | https://pubchem.ncbi.nlm.nih.gov | |
Description | Data deposited in or computed by PubChem | |
InChI |
InChI=1S/C15H26O2/c1-11-5-7-12(8-6-11)15(4)10-9-13(16)14(2,3)17-15/h5,12-13,16H,6-10H2,1-4H3 | |
Details | Computed by InChI 1.0.6 (PubChem release 2021.05.07) | |
Source | PubChem | |
URL | https://pubchem.ncbi.nlm.nih.gov | |
Description | Data deposited in or computed by PubChem | |
InChI Key |
WJHRAVIQWFQMKF-UHFFFAOYSA-N | |
Details | Computed by InChI 1.0.6 (PubChem release 2021.05.07) | |
Source | PubChem | |
URL | https://pubchem.ncbi.nlm.nih.gov | |
Description | Data deposited in or computed by PubChem | |
Canonical SMILES |
CC1=CCC(CC1)C2(CCC(C(O2)(C)C)O)C | |
Details | Computed by OEChem 2.3.0 (PubChem release 2021.05.07) | |
Source | PubChem | |
URL | https://pubchem.ncbi.nlm.nih.gov | |
Description | Data deposited in or computed by PubChem | |
Molecular Formula |
C15H26O2 | |
Details | Computed by PubChem 2.1 (PubChem release 2021.05.07) | |
Source | PubChem | |
URL | https://pubchem.ncbi.nlm.nih.gov | |
Description | Data deposited in or computed by PubChem | |
DSSTOX Substance ID |
DTXSID90945219 | |
Record name | 2,2,6-Trimethyl-6-(4-methylcyclohex-3-en-1-yl)oxan-3-ol | |
Source | EPA DSSTox | |
URL | https://comptox.epa.gov/dashboard/DTXSID90945219 | |
Description | DSSTox provides a high quality public chemistry resource for supporting improved predictive toxicology. | |
Molecular Weight |
238.37 g/mol | |
Details | Computed by PubChem 2.1 (PubChem release 2021.05.07) | |
Source | PubChem | |
URL | https://pubchem.ncbi.nlm.nih.gov | |
Description | Data deposited in or computed by PubChem | |
CAS No. |
58437-68-6, 22567-36-8 | |
Record name | 2H-Pyran-3-ol, tetrahydro-2,2,6-trimethyl-6-(4-methyl-3-cyclohexen-1-yl)- | |
Source | CAS Common Chemistry | |
URL | https://commonchemistry.cas.org/detail?cas_rn=58437-68-6 | |
Description | CAS Common Chemistry is an open community resource for accessing chemical information. Nearly 500,000 chemical substances from CAS REGISTRY cover areas of community interest, including common and frequently regulated chemicals, and those relevant to high school and undergraduate chemistry classes. This chemical information, curated by our expert scientists, is provided in alignment with our mission as a division of the American Chemical Society. | |
Explanation | The data from CAS Common Chemistry is provided under a CC-BY-NC 4.0 license, unless otherwise stated. | |
Record name | Tetrahydro-2,2,6-trimethyl-6-(4-methyl-3-cyclohexen-1-yl)-2H-pyran-3-ol | |
Source | ChemIDplus | |
URL | https://pubchem.ncbi.nlm.nih.gov/substance/?source=chemidplus&sourceid=0058437686 | |
Description | ChemIDplus is a free, web search system that provides access to the structure and nomenclature authority files used for the identification of chemical substances cited in National Library of Medicine (NLM) databases, including the TOXNET system. | |
Record name | 2,2,6-Trimethyl-6-(4-methylcyclohex-3-en-1-yl)oxan-3-ol | |
Source | EPA DSSTox | |
URL | https://comptox.epa.gov/dashboard/DTXSID90945219 | |
Description | DSSTox provides a high quality public chemistry resource for supporting improved predictive toxicology. | |
Record name | Tetrahydro-2,2,6-trimethyl-6-(4-methyl-3-cyclohexen-1-yl)-2H-pyran-3-ol | |
Source | European Chemicals Agency (ECHA) | |
URL | https://echa.europa.eu/substance-information/-/substanceinfo/100.055.662 | |
Description | The European Chemicals Agency (ECHA) is an agency of the European Union which is the driving force among regulatory authorities in implementing the EU's groundbreaking chemicals legislation for the benefit of human health and the environment as well as for innovation and competitiveness. | |
Explanation | Use of the information, documents and data from the ECHA website is subject to the terms and conditions of this Legal Notice, and subject to other binding limitations provided for under applicable law, the information, documents and data made available on the ECHA website may be reproduced, distributed and/or used, totally or in part, for non-commercial purposes provided that ECHA is acknowledged as the source: "Source: European Chemicals Agency, http://echa.europa.eu/". Such acknowledgement must be included in each copy of the material. ECHA permits and encourages organisations and individuals to create links to the ECHA website under the following cumulative conditions: Links can only be made to webpages that provide a link to the Legal Notice page. | |
Record name | alpha-Bisabolol oxide A | |
Source | Human Metabolome Database (HMDB) | |
URL | http://www.hmdb.ca/metabolites/HMDB0038196 | |
Description | The Human Metabolome Database (HMDB) is a freely available electronic database containing detailed information about small molecule metabolites found in the human body. | |
Explanation | HMDB is offered to the public as a freely available resource. Use and re-distribution of the data, in whole or in part, for commercial purposes requires explicit permission of the authors and explicit acknowledgment of the source material (HMDB) and the original publication (see the HMDB citing page). We ask that users who download significant portions of the database cite the HMDB paper in any resulting publications. | |
Retrosynthesis Analysis
AI-Powered Synthesis Planning: Our tool employs the Template_relevance Pistachio, Template_relevance Bkms_metabolic, Template_relevance Pistachio_ringbreaker, Template_relevance Reaxys, Template_relevance Reaxys_biocatalysis model, leveraging a vast database of chemical reactions to predict feasible synthetic routes.
One-Step Synthesis Focus: Specifically designed for one-step synthesis, it provides concise and direct routes for your target compounds, streamlining the synthesis process.
Accurate Predictions: Utilizing the extensive PISTACHIO, BKMS_METABOLIC, PISTACHIO_RINGBREAKER, REAXYS, REAXYS_BIOCATALYSIS database, our tool offers high-accuracy predictions, reflecting the latest in chemical research and data.
Strategy Settings
Precursor scoring | Relevance Heuristic |
---|---|
Min. plausibility | 0.01 |
Model | Template_relevance |
Template Set | Pistachio/Bkms_metabolic/Pistachio_ringbreaker/Reaxys/Reaxys_biocatalysis |
Top-N result to add to graph | 6 |
Feasible Synthetic Routes
Q1: How does bisabolol oxide A exert its anti-inflammatory effects?
A1: While the precise mechanism remains under investigation, research suggests that this compound may inhibit key inflammatory pathways. Studies have demonstrated its ability to selectively inhibit cyclooxygenase-2 (COX-2), an enzyme involved in prostaglandin synthesis, which plays a crucial role in inflammation. []
Q2: Does this compound impact other biological processes relevant to inflammation?
A2: Yes, research suggests this compound might also influence other inflammatory processes. For instance, it has shown potential in reducing the production of pro-inflammatory cytokines, further contributing to its anti-inflammatory profile.
Q3: What is the molecular formula and weight of this compound?
A3: this compound has the molecular formula C15H26O2 and a molecular weight of 238.37 g/mol.
Q4: Is there spectroscopic data available to characterize this compound?
A4: Yes, this compound has been characterized using various spectroscopic techniques, including Gas Chromatography-Mass Spectrometry (GC-MS). GC-MS analysis provides valuable information regarding its fragmentation pattern and helps confirm its identity. [, , , , ]
Q5: How does the stability of this compound vary with different drying methods?
A5: Drying methods significantly impact the preservation of this compound in chamomile. Studies revealed that solar drying helps maintain a higher content of this compound compared to other methods like sun drying or microwave drying. [, ]
Q6: How do different extraction methods influence the yield and quality of this compound?
A6: Various extraction methods yield different quantities and qualities of this compound from chamomile. For instance, pilot plant extraction methods yielded a higher percentage of this compound compared to microwave or laboratory-scale methods. []
Q7: Does this compound exhibit any catalytic properties?
A7: Current research primarily focuses on the biological activities of this compound. There is limited information available regarding its catalytic properties and applications.
Q8: Have there been computational studies conducted on this compound?
A8: Yes, molecular docking studies have been conducted to understand how this compound interacts with target proteins, like AChE. These studies provide insights into its binding mode and potential mechanism of action. []
Q9: Does modifying the structure of this compound affect its biological activity?
A9: While specific SAR studies on this compound are limited within the provided research, it's important to note that structural modifications often influence a molecule's activity. Even slight changes can alter its binding affinity, potency, and selectivity towards specific targets.
Q10: What is being done to improve the stability and delivery of this compound in formulations?
A10: Research explores various approaches to enhance this compound's stability and delivery. Microencapsulation with chitosan, for example, has demonstrated success in improving its stability and enabling controlled release. []
Q11: Is there information available on the safety and regulatory aspects of this compound?
A11: While the provided research focuses on this compound's chemical composition and biological activity, further investigation is crucial to thoroughly assess its safety, toxicology, and adhere to SHE regulations for potential applications.
Q12: Are there specific drug delivery or analytical techniques tailored for this compound?
A12: Research continues to explore advanced delivery systems and analytical techniques for this compound. This includes optimizing existing methods like GC-MS and HPLC to enhance sensitivity, accuracy, and enable comprehensive characterization. [, ]
Q13: What is the environmental impact of this compound production and use?
A13: Research exploring the environmental impact of this compound is crucial. This includes investigating sustainable extraction methods, assessing its biodegradability, and exploring potential alternatives with lower environmental footprints. [, , ]
Q14: How has research on this compound evolved, and what are its future directions?
A14: Research on this compound has progressed from identifying it as a key chamomile constituent to exploring its diverse biological activities. Future research may involve a multidisciplinary approach, integrating computational modeling, targeted drug delivery, and in-depth safety and efficacy studies. [, ]
Q15: How does the geographical location of chamomile cultivation affect the this compound content?
A15: Studies revealed that chamomile cultivated in different geographical locations exhibits variations in this compound content. For example, chamomile from certain regions in Iran demonstrated higher this compound content compared to others. [, ]
Q16: Does the time of day impact the yield and composition of chamomile essential oil, including this compound?
A16: Yes, research indicates that the essential oil content and composition, including this compound, fluctuate throughout the day. The highest essential oil percentage was observed in chamomile flowers harvested between 10:00 am and 12:00 pm. []
Q17: Do different chamomile cultivars exhibit variations in this compound content?
A17: Yes, significant variations in this compound content are observed among different chamomile cultivars. Some cultivars, like 'CIM-Sammohak,' exhibited higher this compound content compared to others like 'CIM-Ujjwala.' []
Q18: How do agricultural practices like fertilization impact the this compound content in chamomile?
A18: Agricultural practices, particularly fertilization, significantly influence the this compound content. For instance, the application of organic fertilizers, such as vermicompost and zeolite, led to an increase in the percentage of this compound in chamomile essential oil. []
Avertissement et informations sur les produits de recherche in vitro
Veuillez noter que tous les articles et informations sur les produits présentés sur BenchChem sont destinés uniquement à des fins informatives. Les produits disponibles à l'achat sur BenchChem sont spécifiquement conçus pour des études in vitro, qui sont réalisées en dehors des organismes vivants. Les études in vitro, dérivées du terme latin "in verre", impliquent des expériences réalisées dans des environnements de laboratoire contrôlés à l'aide de cellules ou de tissus. Il est important de noter que ces produits ne sont pas classés comme médicaments et n'ont pas reçu l'approbation de la FDA pour la prévention, le traitement ou la guérison de toute condition médicale, affection ou maladie. Nous devons souligner que toute forme d'introduction corporelle de ces produits chez les humains ou les animaux est strictement interdite par la loi. Il est essentiel de respecter ces directives pour assurer la conformité aux normes légales et éthiques en matière de recherche et d'expérimentation.