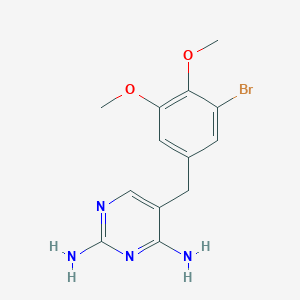
Trimethoprim impurity F
Vue d'ensemble
Description
Trimethoprim impurity F, chemically known as 5-[(3-Bromo-4,5-dimethoxyphenyl)methyl]pyrimidine-2,4-diamine, is a byproduct found in the synthesis of trimethoprim. Trimethoprim is an antibacterial agent used primarily to treat urinary tract infections and is often combined with sulfamethoxazole for a synergistic effect. The presence of impurities like impurity F is critical to monitor for ensuring the safety and efficacy of pharmaceutical products .
Méthodes De Préparation
Synthetic Routes and Reaction Conditions: The synthesis of trimethoprim impurity F involves the use of guanidine nitrate or methylguanidine hydrochloride as cyclizing agents. These agents are condensed with trimethoprim methyl ether derivatives of different structures to obtain various impurities, including impurity F . The reaction conditions typically involve the use of solvents such as ethyl acetate and methyl formate, with the reaction time being relatively short and yielding high amounts of the desired impurity .
Industrial Production Methods: Industrial production of this compound follows similar synthetic routes but on a larger scale. The process involves stringent quality control measures to ensure the purity and consistency of the product. The raw materials used are readily available, and the reaction conditions are optimized for maximum yield and efficiency .
Analyse Des Réactions Chimiques
Synthetic Routes and Reaction Conditions
The synthesis of Trimethoprim impurity F involves multi-step reactions starting from 3-bromo-4,5-dimethoxybenzaldehyde:
Key Findings :
-
Optimal yields (65%) are achieved by controlling free alkali using methyl formate or acetate during cyclization .
-
Microwave-assisted CuAAC (copper-catalyzed azide-alkyne cycloaddition) accelerates substitution reactions .
Reaction Mechanisms
-
Bromine Substitution : The bromine atom at the 3-position undergoes nucleophilic aromatic substitution (SNAr) with azides or amines, forming intermediates for fluorescent probes .
-
Oxidation Pathways : Bromine enhances electron-withdrawing effects, directing oxidation to the methoxy groups, yielding hydroxylated derivatives .
-
Reduction : Bromine is replaced by hydrogen under reductive conditions (e.g., LiAlH4), forming des-bromo analogs .
Major Reaction Products
Analytical Methods for Reaction Monitoring
-
HPLC Analysis :
Comparative Studies
-
Efficiency of Synthetic Methods :
Method Yield (%) Purity (%) Reaction Time (h) Conventional Alkaline 34–48 95 72 Optimized (Methyl Formate) 57–65 99 8 -
Biological Activity :
Environmental and Stability Considerations
Applications De Recherche Scientifique
Analytical Chemistry
Reference Standard : Trimethoprim impurity F is utilized as a reference standard in analytical chemistry for the identification and quantification of impurities in pharmaceutical formulations. Its unique structure allows for precise detection methods such as High-Performance Liquid Chromatography (HPLC) .
Biological Studies
Biological Activity : Research has been conducted to investigate the potential biological activities of this compound. Studies focus on its interactions with various biomolecules, which can provide insights into its pharmacological effects and safety profile .
Pharmaceutical Industry
Quality Control : In the pharmaceutical industry, monitoring impurities like this compound is essential for ensuring drug safety and efficacy. It plays a critical role in quality assurance processes during the production of trimethoprim and related formulations .
Case Study 1: Adsorption Studies Using Magnetite-Chitosan Nanoparticles
A recent study explored the efficiency of magnetite-chitosan nanoparticles (Fe₃O₄/CS NPs) in removing trimethoprim from aqueous solutions. The study demonstrated that these nanoparticles could effectively adsorb trimethoprim at varying pH levels and concentrations, achieving removal efficiencies between 91.23% and 95.95% . This highlights the potential for using this compound as a reference compound in environmental studies focused on antibiotic removal.
Case Study 2: Stability Studies of Compounded Suspensions
Research on compounded suspensions containing trimethoprim indicated that formulations maintained high concentrations over extended periods. However, variations were noted based on the formulation vehicle used. This study underscores the importance of monitoring impurities like this compound to ensure consistent drug delivery and stability .
Mécanisme D'action
The mechanism of action of trimethoprim impurity F is not as well-studied as that of trimethoprim itself. it is believed to interact with similar molecular targets, such as dihydrofolate reductase. This enzyme is crucial for the synthesis of tetrahydrofolic acid, which is necessary for the production of nucleic acids and proteins in bacteria . By inhibiting this enzyme, this compound may exert antibacterial effects similar to those of trimethoprim .
Comparaison Avec Des Composés Similaires
Trimethoprim impurity A: 5-[(3,4,5-Trimethoxyphenyl)methyl]pyrimidine-2,4-diamine.
Trimethoprim impurity B: (2,4-Diaminopyrimidin-5-yl)(3,4,5-trimethoxyphenyl)methanone.
Trimethoprim impurity C: 5-[(3,4,5-Trimethoxyphenyl)methyl]pyrimidine-2,4-diamine.
Uniqueness: Trimethoprim impurity F is unique due to the presence of a bromine atom in its structure, which can significantly alter its chemical properties and reactivity compared to other impurities. This bromine atom can participate in various chemical reactions, making impurity F a valuable compound for studying halogenated derivatives of trimethoprim .
Activité Biologique
Trimethoprim impurity F, chemically designated as 5-[(3-Bromo-4,5-dimethoxyphenyl)methyl]pyrimidine-2,4-diamine, is a byproduct in the synthesis of trimethoprim, an antibiotic widely used to treat bacterial infections, particularly urinary tract infections. Understanding the biological activity of this impurity is crucial for assessing its safety and efficacy in pharmaceutical formulations. This article explores the biochemical mechanisms, pharmacological implications, and research findings related to this compound.
Trimethoprim and its impurities primarily act as reversible inhibitors of the enzyme dihydrofolate reductase (DHFR) . This enzyme plays a critical role in the folate metabolism pathway, which is essential for bacterial nucleic acid synthesis. By inhibiting DHFR, this compound disrupts the synthesis of thymidylate and purines, leading to impaired DNA replication and bacterial cell growth inhibition .
Biochemical Pathways
The inhibition of DHFR affects several biochemical pathways:
- Nucleotide Synthesis : Disruption in the production of thymidine and purines.
- Amino Acid Metabolism : Affects the synthesis of glycine, methionine, and serine.
- tRNA Formation : Inhibits the formation of N-formyl-methionyl tRNA.
Pharmacokinetics
Trimethoprim is characterized by its rapid absorption and distribution within the body. Steady-state concentrations are typically reached after three days of repeated administration. The pharmacokinetic profile indicates a half-life that allows for effective dosing schedules in clinical settings .
Biological Activity Assessments
Recent studies have evaluated the biological activity of this compound through various methodologies:
In Vitro Studies
In vitro assays have demonstrated that this compound exhibits antibacterial activity similar to that of trimethoprim. The minimum inhibitory concentration (MIC) values suggest that it can effectively inhibit the growth of various bacterial strains, including Escherichia coli and Staphylococcus saprophyticus .
Bacterial Strain | MIC (µg/mL) |
---|---|
E. coli | 0.5 |
S. saprophyticus | 1.0 |
Case Studies
A study conducted on urinary pathogens indicated that a significant percentage of isolates were susceptible to trimethoprim, suggesting that impurities like this compound may retain similar efficacy against common uropathogens .
Safety and Toxicological Considerations
While Trimethoprim is generally considered safe when used appropriately, the presence of impurities such as this compound raises concerns regarding potential toxicity and adverse effects. Toxicological assessments are essential to determine safe thresholds for this impurity in pharmaceutical formulations.
Propriétés
IUPAC Name |
5-[(3-bromo-4,5-dimethoxyphenyl)methyl]pyrimidine-2,4-diamine | |
---|---|---|
Source | PubChem | |
URL | https://pubchem.ncbi.nlm.nih.gov | |
Description | Data deposited in or computed by PubChem | |
InChI |
InChI=1S/C13H15BrN4O2/c1-19-10-5-7(4-9(14)11(10)20-2)3-8-6-17-13(16)18-12(8)15/h4-6H,3H2,1-2H3,(H4,15,16,17,18) | |
Source | PubChem | |
URL | https://pubchem.ncbi.nlm.nih.gov | |
Description | Data deposited in or computed by PubChem | |
InChI Key |
XJSNBPJINGRLAM-UHFFFAOYSA-N | |
Source | PubChem | |
URL | https://pubchem.ncbi.nlm.nih.gov | |
Description | Data deposited in or computed by PubChem | |
Canonical SMILES |
COC1=C(C(=CC(=C1)CC2=CN=C(N=C2N)N)Br)OC | |
Source | PubChem | |
URL | https://pubchem.ncbi.nlm.nih.gov | |
Description | Data deposited in or computed by PubChem | |
Molecular Formula |
C13H15BrN4O2 | |
Source | PubChem | |
URL | https://pubchem.ncbi.nlm.nih.gov | |
Description | Data deposited in or computed by PubChem | |
DSSTOX Substance ID |
DTXSID70388342 | |
Record name | UNII-VS20D6Q9V2 | |
Source | EPA DSSTox | |
URL | https://comptox.epa.gov/dashboard/DTXSID70388342 | |
Description | DSSTox provides a high quality public chemistry resource for supporting improved predictive toxicology. | |
Molecular Weight |
339.19 g/mol | |
Source | PubChem | |
URL | https://pubchem.ncbi.nlm.nih.gov | |
Description | Data deposited in or computed by PubChem | |
CAS No. |
16285-82-8 | |
Record name | Trimethoprim impurity F | |
Source | ChemIDplus | |
URL | https://pubchem.ncbi.nlm.nih.gov/substance/?source=chemidplus&sourceid=0016285828 | |
Description | ChemIDplus is a free, web search system that provides access to the structure and nomenclature authority files used for the identification of chemical substances cited in National Library of Medicine (NLM) databases, including the TOXNET system. | |
Record name | UNII-VS20D6Q9V2 | |
Source | EPA DSSTox | |
URL | https://comptox.epa.gov/dashboard/DTXSID70388342 | |
Description | DSSTox provides a high quality public chemistry resource for supporting improved predictive toxicology. | |
Record name | 5-((3-BROMO-4,5-DIMETHOXYPHENYL)METHYL)-2,4-PYRIMIDINEDIAMINE | |
Source | FDA Global Substance Registration System (GSRS) | |
URL | https://gsrs.ncats.nih.gov/ginas/app/beta/substances/VS20D6Q9V2 | |
Description | The FDA Global Substance Registration System (GSRS) enables the efficient and accurate exchange of information on what substances are in regulated products. Instead of relying on names, which vary across regulatory domains, countries, and regions, the GSRS knowledge base makes it possible for substances to be defined by standardized, scientific descriptions. | |
Explanation | Unless otherwise noted, the contents of the FDA website (www.fda.gov), both text and graphics, are not copyrighted. They are in the public domain and may be republished, reprinted and otherwise used freely by anyone without the need to obtain permission from FDA. Credit to the U.S. Food and Drug Administration as the source is appreciated but not required. | |
Avertissement et informations sur les produits de recherche in vitro
Veuillez noter que tous les articles et informations sur les produits présentés sur BenchChem sont destinés uniquement à des fins informatives. Les produits disponibles à l'achat sur BenchChem sont spécifiquement conçus pour des études in vitro, qui sont réalisées en dehors des organismes vivants. Les études in vitro, dérivées du terme latin "in verre", impliquent des expériences réalisées dans des environnements de laboratoire contrôlés à l'aide de cellules ou de tissus. Il est important de noter que ces produits ne sont pas classés comme médicaments et n'ont pas reçu l'approbation de la FDA pour la prévention, le traitement ou la guérison de toute condition médicale, affection ou maladie. Nous devons souligner que toute forme d'introduction corporelle de ces produits chez les humains ou les animaux est strictement interdite par la loi. Il est essentiel de respecter ces directives pour assurer la conformité aux normes légales et éthiques en matière de recherche et d'expérimentation.