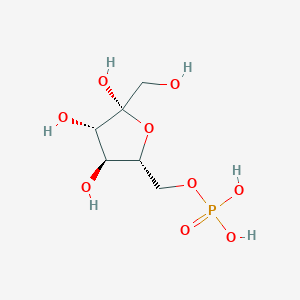
beta-D-Fructose 6-phosphate
Vue d'ensemble
Description
Beta-D-fructose 6-phosphate (β-D-F6P) is a phosphorylated hexose sugar critical to central metabolic pathways, including glycolysis, gluconeogenesis, and the biosynthesis of polysaccharides and nucleotide sugars . Structurally, it is a six-carbon ketose sugar with a phosphate group esterified at the 6th carbon (). Its open-chain and furanose forms participate in enzymatic reactions, such as those catalyzed by phosphofructokinase-1 (PFK1) and fructose-1,6-bisphosphatase (FBPase), which regulate carbon flux between glycolysis and gluconeogenesis . β-D-F6P also serves as a precursor for starch, sucrose, and galactose metabolism , and its accumulation correlates with liver regeneration via enhanced glycolysis .
Activité Biologique
Beta-D-Fructose 6-phosphate (β-D-F6P) is an important metabolite in carbohydrate metabolism, acting as a substrate in various biochemical pathways. This article explores its biological activity, enzymatic interactions, and implications in metabolic processes.
Chemical Structure and Properties
- Chemical Formula : C₆H₁₃O₉P
- Molecular Weight : 260.1358 g/mol
- Anomers : β-D-F6P is one of two anomers of fructose 6-phosphate, the other being alpha-D-fructose 6-phosphate (α-D-F6P).
Enzymatic Functions
β-D-F6P serves as a substrate for several key enzymes involved in metabolic pathways:
Enzyme | Function |
---|---|
Fructose-1,6-bisphosphatase | Converts β-D-F6P to fructose-1,6-bisphosphate |
Pyruvate kinase (isozymes R/L) | Catalyzes the conversion of phosphoenolpyruvate to pyruvate |
Hexokinase (type I) | Phosphorylates glucose to glucose-6-phosphate |
Fructose-bisphosphate aldolase A | Involved in the cleavage of fructose-bisphosphate |
Glyceraldehyde-3-phosphate dehydrogenase | Catalyzes the oxidation of glyceraldehyde-3-phosphate |
These enzymes highlight the role of β-D-F6P in glycolysis and gluconeogenesis.
1. Glycolytic Pathway
β-D-F6P is a crucial intermediate in the glycolytic pathway, where it is converted to fructose-1,6-bisphosphate by phosphofructokinase (PFK). This reaction is a major regulatory step in glycolysis, influencing energy production under varying physiological conditions. PFK activity is notably enhanced by fructose-2,6-bisphosphate (F2,6BP), which is synthesized from β-D-F6P. This reciprocal relationship between β-D-F6P and F2,6BP underscores its significance in cellular energy metabolism .
2. Glucocorticoid Activation
Recent studies have indicated that β-D-F6P can substitute for glucose-6-phosphate (G6P) in glucocorticoid activation processes. In particular, it interacts with hexose-6-phosphate dehydrogenase (H6PD) within the endoplasmic reticulum, facilitating local glucocorticoid activation through NADPH generation. This mechanism highlights its potential role in metabolic syndrome pathogenesis linked to fructose consumption and glucocorticoid activity .
3. Regulatory Mechanisms
The regulation of β-D-F6P levels is critical for maintaining metabolic homeostasis. Hormonal influences, such as those from glucagon and insulin, modulate the synthesis and degradation pathways involving β-D-F6P. For instance, glucagon promotes the phosphorylation of PFK, reducing its affinity for F2,6BP and subsequently lowering glycolytic flux .
Case Study 1: Metabolic Syndrome Implications
A study examined the role of β-D-F6P in white adipose tissue concerning glucocorticoid activation. The findings suggested that increased levels of β-D-F6P were associated with enhanced glucocorticoid activation via H6PD, potentially contributing to the development of metabolic syndrome through altered lipid metabolism and insulin sensitivity .
Case Study 2: Enzyme Interaction Dynamics
Research has shown that β-D-F6P acts as a substrate for various enzymes involved in carbohydrate metabolism. For example, it has been demonstrated that phosphomannose isomerase utilizes β-D-F6P as a substrate, indicating its broader involvement in glycan biosynthesis pathways .
Applications De Recherche Scientifique
Metabolic Role and Enzymatic Interactions
β-D-F6P serves as an important substrate for several key enzymes involved in glycolysis and gluconeogenesis. Notably, it is a substrate for:
- Fructose-1,6-bisphosphatase : Involved in gluconeogenesis, converting fructose-1,6-bisphosphate to fructose-6-phosphate.
- Hexokinase : Catalyzes the phosphorylation of glucose and fructose.
- Pyruvate Kinase : Plays a role in the final steps of glycolysis.
These interactions highlight β-D-F6P's central role in carbohydrate metabolism and energy production .
Role in Glucocorticoid Activation
Recent studies have shown that β-D-F6P can substitute for glucose-6-phosphate (G6P) in maintaining the activity of 11β-hydroxysteroid dehydrogenase type 1 (11β-HSD1). This enzyme is crucial for the activation of glucocorticoids, which are implicated in metabolic syndrome pathogenesis. The ability of β-D-F6P to promote glucocorticoid activation suggests its potential role in metabolic disorders .
Applications in Microbial Metabolism
In microbiology, β-D-F6P has been studied for its role in the metabolism of various microorganisms. For instance:
- Bifidobacterium spp. : Research indicates that strains with acquired bile resistance exhibit altered membrane-associated protein profiles, including increased activity of fructose-6-phosphate phosphoketolase. This enzyme is essential for carbohydrate catabolism in these bacteria, enhancing glucose consumption rates .
Structural Studies and Binding Affinities
Structural studies have provided insights into how β-D-F6P interacts with phosphofructokinase (PFK), a key regulatory enzyme in glycolysis. Mutational analyses have demonstrated that specific residues within PFK are critical for binding β-D-F6P, influencing both catalytic activity and allosteric regulation .
Case Study 1: Glucocorticoid Activation Mechanism
A study demonstrated that β-D-F6P effectively maintains the reductase activity of 11β-HSD1 under conditions where G6P is absent. This finding suggests that β-D-F6P could be targeted for therapeutic strategies aimed at metabolic syndrome management .
Case Study 2: Microbial Resistance Mechanisms
In Bifidobacterium bifidum, the enhanced activity of fructose-6-phosphate phosphoketolase was linked to increased bile resistance. This adaptation underscores the importance of β-D-F6P in microbial survival and metabolic flexibility under stress conditions .
Data Table: Enzymatic Interactions with β-D-F6P
Enzyme | Reaction Type | Role of β-D-F6P |
---|---|---|
Fructose-1,6-bisphosphatase | Gluconeogenesis | Substrate for conversion to F6P |
Hexokinase | Glycolysis | Phosphorylation of F6P |
Pyruvate Kinase | Glycolysis | Final step conversion |
Fructose-bisphosphate aldolase | Glycolysis | Conversion involving F6P |
Analyse Des Réactions Chimiques
From Glucose 6-Phosphate
Enzyme : Phosphoglucose isomerase (EC 5.3.1.9)
Reaction :
Role : Reversible isomerization critical for glycolysis entry .
From Mannose 6-Phosphate
Enzyme : Mannose-6-phosphate isomerase (EC 5.3.1.8)
Reaction :
Role : Connects mannose metabolism to glycolysis .
From Sorbitol 6-Phosphate
Enzyme : Sorbitol-6-phosphate 2-dehydrogenase (EC 1.1.1.140)
Reaction :
Role : Links polyol metabolism to glycolysis .
Phosphorylation to Fructose 1,6-Bisphosphate
Enzyme : Phosphofructokinase (EC 2.7.1.11)
Reaction :
Role : Commits glucose to glycolysis; regulated by ATP/AMP levels .
Hydrolysis in Gluconeogenesis
Enzyme : Fructose-1,6-bisphosphatase (FBPase; EC 3.1.3.11)
Reaction :
Mechanism :
-
SN2 nucleophilic attack : A hydroxide ion (activated by Mg²⁺ and Asp74 ) displaces the C1 phosphate group.
-
Transition state : Pentavalent phosphate intermediate stabilized by three divalent metal ions (Mg²⁺/Mn²⁺) .
Transaldolase-Mediated Transfer
Enzyme : Transaldolase (EC 2.2.1.2)
Reaction :
Role : Generates precursors for nucleotide synthesis .
Catalytic Residues and Metal Coordination in FBPase
Residue | Role |
---|---|
Asp74 | Activates water via proton abstraction |
Glu98 | Polarizes nucleophilic water |
Mg²⁺/Mn²⁺ | Stabilizes negative charges in transition state |
Mechanistic Steps :
-
Substrate binding : Fructose 1,6-bisphosphate coordinates with three metal ions.
-
Nucleophilic attack : Asp74 deprotonates water, generating a hydroxide ion for SN2 displacement of the C1 phosphate .
-
Product release : Phosphate and β-D-F6P dissociate, regenerating the enzyme .
Metabolic Pathways
-
Glycolysis : Converted to fructose 1,6-bisphosphate via phosphofructokinase .
-
Gluconeogenesis : Generated from fructose 1,6-bisphosphate via FBPase .
-
Pentose Phosphate Pathway : Produced via transaldolase activity .
Regulatory Interactions
Enzyme | Activators | Inhibitors |
---|---|---|
Phosphofructokinase | AMP, Fructose 2,6-bisphosphate | ATP, Citrate |
FBPase | Citrate | AMP, Fructose 2,6-bisphosphate |
Cross-regulation : Fructose 2,6-bisphosphate reciprocally regulates phosphofructokinase (activates) and FBPase (inhibits), ensuring pathway coordination .
Q & A
Basic Research Questions
Q. What is the role of β-D-Fructose 6-phosphate in central carbon metabolism, and how can its flux be experimentally tracked?
β-D-F6P is a critical intermediate in glycolysis, gluconeogenesis, and the pentose phosphate pathway (PPP). In glycolysis, glucose-6-phosphate isomerase (GPI) converts glucose-6-phosphate to β-D-F6P, which is then phosphorylated by phosphofructokinase (PFK) to fructose-1,6-bisphosphate . In gluconeogenesis, fructose-1,6-bisphosphatase (FBPase) catalyzes the reverse reaction. To track its metabolic flux, researchers use isotopic labeling (e.g., ¹³C-glucose) combined with mass spectrometry or nuclear magnetic resonance (NMR) to trace carbon redistribution through downstream metabolites like glyceraldehyde 3-phosphate or PPP-derived ribose .
Q. What methodologies are recommended for quantifying β-D-Fructose 6-phosphate in cellular extracts?
Enzymatic coupled assays are widely used. For example, β-D-F6P can be quantified by coupling its conversion to glucose-6-phosphate via GPI, followed by glucose-6-phosphate dehydrogenase (G6PDH)-mediated reduction of NADP⁺ to NADPH. NADPH absorbance at 340 nm is measured spectrophotometrically . Alternatively, high-performance liquid chromatography (HPLC) with refractive index or tandem mass spectrometry (LC-MS/MS) provides higher specificity for complex biological matrices .
Advanced Research Questions
Q. How do allosteric regulators and post-translational modifications influence PFK affinity for β-D-Fructose 6-phosphate?
PFK activity is modulated by fructose-1,6-bisphosphate (F1,6BP), ATP, and AMP. For example, F1,6BP enhances PFK’s affinity for β-D-F6P, while glucagon signaling (via cAMP-PKA) reduces this affinity by phosphorylating PFK . Methodologically, kinetic assays under varying substrate concentrations (e.g., F6P ± F1,6BP) and site-directed mutagenesis of PFK phosphorylation sites can dissect regulatory mechanisms .
Q. What experimental approaches distinguish the biological roles of β-D-F6P from its α-anomer?
The β-anomer is the predominant form in glycolysis, while the α-anomer may accumulate under specific conditions (e.g., enzyme deficiencies). To differentiate their roles:
- Use chiral chromatography (e.g., HPLC with a chiral column) or ³¹P-NMR to resolve anomers .
- Compare enzyme kinetics (e.g., GPI or PFK activity) with purified α- vs. β-D-F6P substrates .
Q. How can metabolic engineering elucidate β-D-F6P’s role in stress responses in non-model organisms?
In archaea or plant systems, CRISPR/Cas9-mediated knockout of GPI or PFK isoforms can disrupt β-D-F6P production. For example, in Malus (apple), RNA-seq of PFK1/FBPase mutants revealed altered expression of stress-responsive genes linked to fructose-6-phosphate accumulation . Flux balance analysis (FBA) models can further predict metabolic rerouting under genetic or environmental perturbations .
Q. How should researchers resolve contradictions in reported kinetic parameters for β-D-F6P-dependent enzymes?
Discrepancies often arise from assay conditions (e.g., pH, ionic strength, or presence of SDS). For PFK, compare kinetic data obtained in:
- In vitro purified systems (e.g., recombinant PFK ± F1,6BP) .
- Cellular lysates with physiological metabolite concentrations . Standardize assays using protocols from repositories like BRENDA or MetaCyc .
Q. Methodological Considerations Table
Comparaison Avec Des Composés Similaires
Comparison with Similar Compounds
Beta-D-fructose 6-phosphate shares structural and functional similarities with other phosphorylated hexoses, but key distinctions define its unique roles. Below is a detailed analysis:
Structural Comparisons
Beta-D-Fructose 1,6-Bisphosphate (β-D-F1,6BP) :
β-D-F6P and β-D-F1,6BP differ in phosphorylation state (one vs. two phosphate groups) and structure. β-D-F6P retains a ring structure, while β-D-F1,6BP adopts a linear conformation, making it distinct in enzyme binding and metabolic partitioning . The absence of a ring in β-D-F1,6BP reduces its similarity to β-D-F6P (structural similarity <0.4 in graph-based analyses) .Alpha-D-Glucose 6-Phosphate (α-D-G6P) :
Both compounds are hexose-6-phosphates but differ in stereochemistry (glucose vs. fructose) and isomerization state. α-D-G6P is an aldose, while β-D-F6P is a ketose. Despite this, their ring structures confer moderate similarity (similarity index >0.6), enabling shared roles in glycolysis and the pentose phosphate pathway (PPP) .- Mannose 6-Phosphate (M6P): M6P is an epimer of α-D-G6P at the C2 position. Unlike β-D-F6P, M6P is primarily associated with lysosomal enzyme targeting and glycosylation rather than energy production .
Metabolic Roles and Pathway Integration
- Glycolysis vs. Gluconeogenesis: β-D-F6P is interconverted with β-D-F1,6BP via PFK1 (glycolysis) and FBPase (gluconeogenesis). While β-D-F1,6BP is a committed glycolytic intermediate, β-D-F6P serves as a node for branching into the PPP or polysaccharide synthesis .
- Energy and Biosynthesis :
In Phakopsora pachyrhizi, β-D-F6P levels correlate with fungal energy production during infection, whereas β-D-F1,6BP is downregulated under nutrient stress . In plants, β-D-F6P accumulation drives polysaccharide synthesis in Polygonatum odoratum rhizomes .
Regulatory and Disease Contexts
- IUGR Model: β-D-F6P, α-D-G6P, and M6P are downregulated in intrauterine growth restriction (IUGR), indicating their sensitivity to metabolic dysregulation in fructose/mannose metabolism .
- Liver Regeneration :
β-D-F6P elevation in hepatocytes enhances glycolysis via the JAK2/STAT3/HK2 pathway, a response absent in M6P or α-D-G6P . - Enzyme Specificity : Transaldolase 1 (TALDO1) in the PPP preferentially processes β-D-F6P and D-glyceraldehyde 3-phosphate, unlike fructose-1,6-bisphosphate aldolase, which acts on β-D-F1,6BP .
Propriétés
IUPAC Name |
[(2R,3S,4S,5R)-3,4,5-trihydroxy-5-(hydroxymethyl)oxolan-2-yl]methyl dihydrogen phosphate | |
---|---|---|
Source | PubChem | |
URL | https://pubchem.ncbi.nlm.nih.gov | |
Description | Data deposited in or computed by PubChem | |
InChI |
InChI=1S/C6H13O9P/c7-2-6(10)5(9)4(8)3(15-6)1-14-16(11,12)13/h3-5,7-10H,1-2H2,(H2,11,12,13)/t3-,4-,5+,6-/m1/s1 | |
Source | PubChem | |
URL | https://pubchem.ncbi.nlm.nih.gov | |
Description | Data deposited in or computed by PubChem | |
InChI Key |
BGWGXPAPYGQALX-ARQDHWQXSA-N | |
Source | PubChem | |
URL | https://pubchem.ncbi.nlm.nih.gov | |
Description | Data deposited in or computed by PubChem | |
Canonical SMILES |
C(C1C(C(C(O1)(CO)O)O)O)OP(=O)(O)O | |
Source | PubChem | |
URL | https://pubchem.ncbi.nlm.nih.gov | |
Description | Data deposited in or computed by PubChem | |
Isomeric SMILES |
C([C@@H]1[C@H]([C@@H]([C@](O1)(CO)O)O)O)OP(=O)(O)O | |
Source | PubChem | |
URL | https://pubchem.ncbi.nlm.nih.gov | |
Description | Data deposited in or computed by PubChem | |
Molecular Formula |
C6H13O9P | |
Source | PubChem | |
URL | https://pubchem.ncbi.nlm.nih.gov | |
Description | Data deposited in or computed by PubChem | |
DSSTOX Substance ID |
DTXSID90889361 | |
Record name | D-Fructose, 6-(dihydrogen phosphate) | |
Source | EPA DSSTox | |
URL | https://comptox.epa.gov/dashboard/DTXSID90889361 | |
Description | DSSTox provides a high quality public chemistry resource for supporting improved predictive toxicology. | |
Molecular Weight |
260.14 g/mol | |
Source | PubChem | |
URL | https://pubchem.ncbi.nlm.nih.gov | |
Description | Data deposited in or computed by PubChem | |
Physical Description |
Solid | |
Record name | Beta-D-Fructose 6-phosphate | |
Source | Human Metabolome Database (HMDB) | |
URL | http://www.hmdb.ca/metabolites/HMDB0003971 | |
Description | The Human Metabolome Database (HMDB) is a freely available electronic database containing detailed information about small molecule metabolites found in the human body. | |
Explanation | HMDB is offered to the public as a freely available resource. Use and re-distribution of the data, in whole or in part, for commercial purposes requires explicit permission of the authors and explicit acknowledgment of the source material (HMDB) and the original publication (see the HMDB citing page). We ask that users who download significant portions of the database cite the HMDB paper in any resulting publications. | |
CAS No. |
643-13-0 | |
Record name | D-Fructose, 6-(dihydrogen phosphate) | |
Source | EPA DSSTox | |
URL | https://comptox.epa.gov/dashboard/DTXSID90889361 | |
Description | DSSTox provides a high quality public chemistry resource for supporting improved predictive toxicology. | |
Retrosynthesis Analysis
AI-Powered Synthesis Planning: Our tool employs the Template_relevance Pistachio, Template_relevance Bkms_metabolic, Template_relevance Pistachio_ringbreaker, Template_relevance Reaxys, Template_relevance Reaxys_biocatalysis model, leveraging a vast database of chemical reactions to predict feasible synthetic routes.
One-Step Synthesis Focus: Specifically designed for one-step synthesis, it provides concise and direct routes for your target compounds, streamlining the synthesis process.
Accurate Predictions: Utilizing the extensive PISTACHIO, BKMS_METABOLIC, PISTACHIO_RINGBREAKER, REAXYS, REAXYS_BIOCATALYSIS database, our tool offers high-accuracy predictions, reflecting the latest in chemical research and data.
Strategy Settings
Precursor scoring | Relevance Heuristic |
---|---|
Min. plausibility | 0.01 |
Model | Template_relevance |
Template Set | Pistachio/Bkms_metabolic/Pistachio_ringbreaker/Reaxys/Reaxys_biocatalysis |
Top-N result to add to graph | 6 |
Feasible Synthetic Routes
Avertissement et informations sur les produits de recherche in vitro
Veuillez noter que tous les articles et informations sur les produits présentés sur BenchChem sont destinés uniquement à des fins informatives. Les produits disponibles à l'achat sur BenchChem sont spécifiquement conçus pour des études in vitro, qui sont réalisées en dehors des organismes vivants. Les études in vitro, dérivées du terme latin "in verre", impliquent des expériences réalisées dans des environnements de laboratoire contrôlés à l'aide de cellules ou de tissus. Il est important de noter que ces produits ne sont pas classés comme médicaments et n'ont pas reçu l'approbation de la FDA pour la prévention, le traitement ou la guérison de toute condition médicale, affection ou maladie. Nous devons souligner que toute forme d'introduction corporelle de ces produits chez les humains ou les animaux est strictement interdite par la loi. Il est essentiel de respecter ces directives pour assurer la conformité aux normes légales et éthiques en matière de recherche et d'expérimentation.