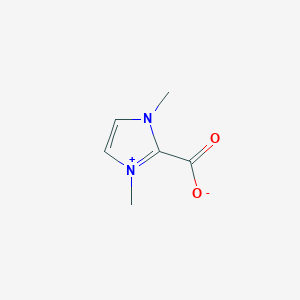
1,3-Dimethylimidazolium-2-carboxylate
Vue d'ensemble
Description
“1,3-Dimethylimidazolium-2-carboxylate” is a compound that is formed as a reaction product resulting from both N-alkylation and C-carboxylation of 1-methylimidazole with dimethyl carbonate . The crystal structure of the zwitterion exhibits π-stacked rings and two-dimensional sheets constructed by hydrogen-bonds from imidazolium-ring hydrogens to the carboxylate group .
Synthesis Analysis
The synthesis of “1,3-Dimethylimidazolium-2-carboxylate” involves the reaction of 1-methylimidazole with dimethyl carbonate, resulting in both N-alkylation and C-carboxylation . This process forms “1,3-Dimethylimidazolium-2-carboxylate” in good yield, rather than the anticipated organic salt, 1,3-dimethylimidazolium methyl carbonate .
Molecular Structure Analysis
The molecular formula of “1,3-Dimethylimidazolium-2-carboxylate” is C6H8N2O2 . The crystal structure of the zwitterion exhibits π-stacked rings and two-dimensional sheets constructed by hydrogen-bonds from imidazolium-ring hydrogens to the carboxylate group .
Chemical Reactions Analysis
“1,3-Dimethylimidazolium-2-carboxylate” is formed as a reaction product resulting from both N-alkylation and C-carboxylation of 1-methylimidazole with dimethyl carbonate .
Physical And Chemical Properties Analysis
The molecular weight of “1,3-Dimethylimidazolium-2-carboxylate” is 140.14 g/mol . It has a Hydrogen Bond Donor Count of 0 and a Hydrogen Bond Acceptor Count of 2 . The compound has a Rotatable Bond Count of 0 . The Exact Mass and Monoisotopic Mass of the compound is 140.058577502 g/mol . The Topological Polar Surface Area of the compound is 48.9 Ų .
Applications De Recherche Scientifique
Ionic Liquid Precursor
1,3-Dimethylimidazolium-2-carboxylate is formed in good yield, rather than the anticipated organic salt, 1,3-dimethylimidazolium methyl carbonate, as the reaction product resulting from both N-alkylation and C-carboxylation of 1-methylimidazole with dimethyl carbonate . This makes it an unexpected synthesis of an ionic liquid precursor .
Carbene-CO2 Adduct
The compound also acts as a carbene-CO2 adduct . The crystal structure of the zwitterion exhibits π-stacked rings and two-dimensional sheets constructed by hydrogen-bonds from imidazolium-ring hydrogens to the carboxylate group .
Efficient Synthesis of Vicinal Diols
1,3-Dimethylimidazolium-2-carboxylate is a zwitterionic salt used for the efficient synthesis of vicinal diols from cyclic carbonates . It showed high activity and excellent selectivity for the preparation of ethylene glycol via the hydrolysis of ethylene carbonate .
Recyclable Catalyst
This compound is a simple halogen-free zwitterionic catalyst that can be reused over six times without obvious loss of catalytic activity . This makes it a valuable tool in green chemistry.
5. Applicable to a Variety of Cyclic Carbonates 1,3-Dimethylimidazolium-2-carboxylate is applicable to a variety of cyclic carbonates for the production of their corresponding vicinal diols with high yields and selectivities .
Preparation of Heterocyclic Carbene Adducts
The synthesis of 1,3-Dimethylimidazolium-2-carboxylate is of interest as a potentially clean, minimal waste, route to prepare ionic liquids, and also for the preparation of heterocyclic carbene adducts without using traditional strong bases .
Propriétés
IUPAC Name |
1,3-dimethylimidazol-1-ium-2-carboxylate | |
---|---|---|
Source | PubChem | |
URL | https://pubchem.ncbi.nlm.nih.gov | |
Description | Data deposited in or computed by PubChem | |
InChI |
InChI=1S/C6H8N2O2/c1-7-3-4-8(2)5(7)6(9)10/h3-4H,1-2H3 | |
Source | PubChem | |
URL | https://pubchem.ncbi.nlm.nih.gov | |
Description | Data deposited in or computed by PubChem | |
InChI Key |
HESZXGSSISDCNI-UHFFFAOYSA-N | |
Source | PubChem | |
URL | https://pubchem.ncbi.nlm.nih.gov | |
Description | Data deposited in or computed by PubChem | |
Canonical SMILES |
CN1C=C[N+](=C1C(=O)[O-])C | |
Source | PubChem | |
URL | https://pubchem.ncbi.nlm.nih.gov | |
Description | Data deposited in or computed by PubChem | |
Molecular Formula |
C6H8N2O2 | |
Source | PubChem | |
URL | https://pubchem.ncbi.nlm.nih.gov | |
Description | Data deposited in or computed by PubChem | |
DSSTOX Substance ID |
DTXSID00467811 | |
Record name | 1,3-Dimethyl-1H-imidazol-3-ium-2-carboxylate | |
Source | EPA DSSTox | |
URL | https://comptox.epa.gov/dashboard/DTXSID00467811 | |
Description | DSSTox provides a high quality public chemistry resource for supporting improved predictive toxicology. | |
Molecular Weight |
140.14 g/mol | |
Source | PubChem | |
URL | https://pubchem.ncbi.nlm.nih.gov | |
Description | Data deposited in or computed by PubChem | |
Product Name |
1,3-Dimethylimidazolium-2-carboxylate | |
CAS RN |
536755-29-0 | |
Record name | 1,3-Dimethyl-1H-imidazol-3-ium-2-carboxylate | |
Source | EPA DSSTox | |
URL | https://comptox.epa.gov/dashboard/DTXSID00467811 | |
Description | DSSTox provides a high quality public chemistry resource for supporting improved predictive toxicology. | |
Record name | 1,3-Dimethylimidazolium-2-carboxylate | |
Source | European Chemicals Agency (ECHA) | |
URL | https://echa.europa.eu/information-on-chemicals | |
Description | The European Chemicals Agency (ECHA) is an agency of the European Union which is the driving force among regulatory authorities in implementing the EU's groundbreaking chemicals legislation for the benefit of human health and the environment as well as for innovation and competitiveness. | |
Explanation | Use of the information, documents and data from the ECHA website is subject to the terms and conditions of this Legal Notice, and subject to other binding limitations provided for under applicable law, the information, documents and data made available on the ECHA website may be reproduced, distributed and/or used, totally or in part, for non-commercial purposes provided that ECHA is acknowledged as the source: "Source: European Chemicals Agency, http://echa.europa.eu/". Such acknowledgement must be included in each copy of the material. ECHA permits and encourages organisations and individuals to create links to the ECHA website under the following cumulative conditions: Links can only be made to webpages that provide a link to the Legal Notice page. | |
Retrosynthesis Analysis
AI-Powered Synthesis Planning: Our tool employs the Template_relevance Pistachio, Template_relevance Bkms_metabolic, Template_relevance Pistachio_ringbreaker, Template_relevance Reaxys, Template_relevance Reaxys_biocatalysis model, leveraging a vast database of chemical reactions to predict feasible synthetic routes.
One-Step Synthesis Focus: Specifically designed for one-step synthesis, it provides concise and direct routes for your target compounds, streamlining the synthesis process.
Accurate Predictions: Utilizing the extensive PISTACHIO, BKMS_METABOLIC, PISTACHIO_RINGBREAKER, REAXYS, REAXYS_BIOCATALYSIS database, our tool offers high-accuracy predictions, reflecting the latest in chemical research and data.
Strategy Settings
Precursor scoring | Relevance Heuristic |
---|---|
Min. plausibility | 0.01 |
Model | Template_relevance |
Template Set | Pistachio/Bkms_metabolic/Pistachio_ringbreaker/Reaxys/Reaxys_biocatalysis |
Top-N result to add to graph | 6 |
Feasible Synthetic Routes
Avertissement et informations sur les produits de recherche in vitro
Veuillez noter que tous les articles et informations sur les produits présentés sur BenchChem sont destinés uniquement à des fins informatives. Les produits disponibles à l'achat sur BenchChem sont spécifiquement conçus pour des études in vitro, qui sont réalisées en dehors des organismes vivants. Les études in vitro, dérivées du terme latin "in verre", impliquent des expériences réalisées dans des environnements de laboratoire contrôlés à l'aide de cellules ou de tissus. Il est important de noter que ces produits ne sont pas classés comme médicaments et n'ont pas reçu l'approbation de la FDA pour la prévention, le traitement ou la guérison de toute condition médicale, affection ou maladie. Nous devons souligner que toute forme d'introduction corporelle de ces produits chez les humains ou les animaux est strictement interdite par la loi. Il est essentiel de respecter ces directives pour assurer la conformité aux normes légales et éthiques en matière de recherche et d'expérimentation.