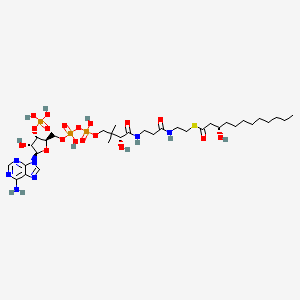
(S)-3-hydroxylauroyl-CoA
- Cliquez sur DEMANDE RAPIDE pour recevoir un devis de notre équipe d'experts.
- Avec des produits de qualité à un prix COMPÉTITIF, vous pouvez vous concentrer davantage sur votre recherche.
Vue d'ensemble
Description
(S)-3-hydroxylauroyl-CoA is a hydroxy fatty acyl-CoA that results from the formal condensation of the thiol group of coenzyme A with the carboxy group of (S)-3-hydroxydodecanoic acid. It has a role as a human metabolite, a Saccharomyces cerevisiae metabolite, an Escherichia coli metabolite and a mouse metabolite. It is a (S)-3-hydroxyacyl-CoA, a 3-hydroxy fatty acyl-CoA and a medium-chain fatty acyl-CoA. It derives from a lauroyl-CoA and a (S)-3-hydroxylauric acid. It is a conjugate acid of a this compound(4-).
Applications De Recherche Scientifique
Biochemical Properties
- Chemical Structure : (S)-3-hydroxylauroyl-CoA is characterized by a hydroxyl group at the 3-position of the lauroyl chain, which influences its reactivity and interaction with enzymes.
- Metabolic Role : It participates in the synthesis and degradation of fatty acids, acting as a substrate for dehydrogenases and other enzymes involved in lipid metabolism .
Metabolic Engineering
Metabolic engineering utilizes this compound to enhance the production of biofuels and biochemicals. By manipulating pathways that involve this compound, researchers can increase yields of desired metabolites.
- Case Study : In a study involving Clostridium tyobutyricum, this compound was shown to be a substrate for specific dehydrogenases, facilitating the conversion of fatty acids into valuable products like butyric acid . This demonstrates its potential in biofuel production.
Drug Development
This compound has implications in drug design due to its role in lipid metabolism. Compounds that mimic or inhibit its activity can be developed into therapeutic agents targeting metabolic disorders.
- Research Insight : Studies have indicated that manipulating pathways involving this compound can influence the synthesis of bioactive lipids, which may lead to novel anti-inflammatory drugs .
Biocatalysis
The enzyme-catalyzed reactions involving this compound are crucial for developing biocatalytic processes that are environmentally friendly and efficient.
- Example : Enzymes such as hydroxylases can utilize this compound to introduce hydroxyl groups into various substrates, enhancing their solubility and reactivity for further chemical transformations .
Data Table: Key Properties and Applications
Property/Application | Description |
---|---|
Chemical Identity | (S)-3-Hydroxydodecanoyl-CoA |
Role in Metabolism | Substrate for dehydrogenases; involved in fatty acid metabolism |
Biotechnological Use | Enhancing biofuel production through metabolic engineering |
Pharmaceutical Potential | Development of drugs targeting lipid metabolism disorders |
Enzymatic Reactions | Acts as a substrate for biocatalytic processes utilizing hydroxylases |
Analyse Des Réactions Chimiques
Metabolic Role in Fatty Acid Elongation
(S)-3-Hydroxylauroyl-CoA serves as an intermediate in the elongation of fatty acids within mitochondria and peroxisomes. Key reactions include:
Dehydration to Enoyl-CoA
-
Catalyzed by 3-hydroxyacyl-CoA dehydratase , the hydroxyl group at the C3 position is removed, forming trans-2-dodecenoyl-CoA .
-
This reaction precedes reduction by trans-2-enoyl-CoA reductase to yield acyl-CoA derivatives for further elongation .
Oxidation to 3-Ketolauroyl-CoA
-
Long-chain-3-hydroxyacyl-CoA dehydrogenase (HADH) oxidizes this compound to 3-ketolauroyl-CoA, coupled with NAD+ reduction to NADH .
S 3 Hydroxylauroyl CoA+NAD+→3 Ketolauroyl CoA+NADH+H+
Enzymatic Hydrolysis
Hydrolysis to Free Acid
-
3-Hydroxyisobutyryl-CoA hydrolase (EHD3) cleaves the thioester bond, releasing (S)-3-hydroxylauric acid and coenzyme A :
S 3 Hydroxylauroyl CoA+H2O→ S 3 Hydroxylauric Acid+CoA SH -
This reaction is critical in valine catabolism and mitochondrial protein biosynthesis .
Thiolytic Cleavage in β-Oxidation
In peroxisomes, 3-ketoacyl-CoA thiolase (POT1) cleaves 3-ketolauroyl-CoA (derived from oxidation) into acetyl-CoA and a shorter acyl-CoA :
3 Ketolauroyl CoA+CoA SH→Decanoyl CoA+Acetyl CoA
This step shortens the fatty acid chain by two carbons, enabling iterative β-oxidation .
Stereochemical Specificity
Enzymes interacting with this compound exhibit strict stereoselectivity:
-
HADH and EHD3 exclusively recognize the S-isomer, ensuring metabolic fidelity .
-
Mutations in these enzymes disrupt lipid metabolism, linking to disorders like HADH deficiency .
Table 1: Key Reactions of this compound
Reaction Type | Enzyme | Substrate | Product(s) | Location |
---|---|---|---|---|
Oxidation | Long-chain-3-hydroxyacyl-CoA dehydrogenase | This compound + NAD+ | 3-Ketolauroyl-CoA + NADH | Mitochondria |
Hydrolysis | 3-Hydroxyisobutyryl-CoA hydrolase | This compound + H2O | (S)-3-Hydroxylauric Acid + CoA-SH | Mitochondria |
Thiolytic Cleavage | 3-Ketoacyl-CoA thiolase | 3-Ketolauroyl-CoA + CoA-SH | Decanoyl-CoA + Acetyl-CoA | Peroxisomes |
Stability and Reactivity
Q & A
Basic Research Questions
Q. What are the standard methodologies for detecting and quantifying (S)-3-hydroxylauroyl-CoA in biological samples?
- Methodological Answer : this compound can be quantified using enzymatic assays coupled with spectrophotometric or chromatographic techniques. For example, enzymatic activity assays for short-chain enoyl-CoA hydratase (EC 4.2.1.150) can be employed, where the dehydration of this compound to trans-2-enoyl-CoA is monitored via absorbance changes at 263 nm . High-performance liquid chromatography (HPLC) or liquid chromatography-mass spectrometry (LC-MS) is recommended for precise quantification, with protocols adapted from studies on structurally similar compounds like (S)-3-hydroxybutyryl-CoA . Protein quantification in enzyme preparations can utilize the Bradford method for sensitivity .
Q. How is this compound synthesized for in vitro studies?
- Methodological Answer : Chemical synthesis typically involves enzymatic acylation of CoA with lauroyl groups, followed by stereospecific hydroxylation at the 3-position. Protocols for synthesizing hydroxyacyl-CoA derivatives often use recombinant acyl-CoA synthetases or thioesterases, as described for related hydroxyacyl-CoAs . Purity is validated via nuclear magnetic resonance (NMR) and LC-MS, with reference to SMILES strings and structural databases .
Q. What experimental controls are critical when studying this compound in metabolic pathways?
- Methodological Answer : Include negative controls (e.g., enzyme inhibitors like sodium arsenite for dehydratases) and substrate-free reactions to rule out non-specific hydrolysis. Use isotopically labeled (e.g., ¹³C or ²H) this compound to track metabolic flux, as demonstrated in studies on β-oxidation intermediates . Ensure protein concentrations are standardized using methods like Lowry or Bradford assays .
Advanced Research Questions
Q. How can conflicting data on the role of this compound in lipid metabolism be resolved?
- Methodological Answer : Discrepancies may arise from differences in model systems (e.g., bacterial vs. mammalian enzymes) or assay conditions (pH, temperature). Perform comparative kinetic analyses using purified enzymes (e.g., short-chain enoyl-CoA hydratase) under standardized conditions . Meta-analyses of transcriptomic and metabolomic datasets can identify context-dependent pathway interactions, as shown in metabolic network models . Controlled variables (e.g., cofactor availability) should be explicitly reported to align with ALCOA+ data integrity criteria .
Q. What strategies are effective for elucidating the structural determinants of enzyme specificity toward this compound?
- Methodological Answer : Use X-ray crystallography or cryo-EM to resolve enzyme-substrate complexes, referencing structural studies on human heart short-chain L-3-hydroxyacyl-CoA dehydrogenase . Site-directed mutagenesis can validate catalytic residues, while molecular dynamics simulations predict substrate binding affinities. Cross-reference systematic enzyme nomenclature (e.g., EC 4.2.1.150) to ensure alignment with IUBMB classifications .
Q. How does this compound participate in crosstalk between fatty acid oxidation and secondary metabolism?
- Methodological Answer : Isotopic tracing with ¹³C-labeled this compound in cell cultures can map its incorporation into polyketide or acylated natural products. Pathway inhibition studies (e.g., CRISPR knockouts of hydratases) combined with metabolomics reveal compensatory mechanisms, as seen in bacteriophage T4 assembly research . Data contradictions (e.g., substrate promiscuity) should be analyzed using iterative qualitative frameworks .
Q. Data Analysis & Interpretation
Q. What statistical approaches are suitable for analyzing time-dependent effects of this compound accumulation?
- Methodological Answer : Longitudinal studies benefit from structural equation modeling (SEM) to assess causal relationships, as applied in studies on metabolic stress . For small sample sizes, non-parametric tests (e.g., Mann-Whitney U) are preferred. Data should adhere to open science principles, with raw datasets archived in repositories like the European Open Science Cloud .
Q. How can researchers address contradictions in substrate specificity across homologous enzymes?
- Methodological Answer : Phylogenetic analysis of enzyme sequences identifies evolutionary divergence points, while in vitro reconstitution assays test functional conservation. For example, compare bacterial crotonases (EC 4.2.1.150) with mammalian homologs using standardized activity assays . Contradictions are minimized by reporting detailed methods (e.g., buffer composition, detection limits) as per good documentation practices .
Q. Experimental Design
Q. What are best practices for designing assays to monitor this compound turnover in real time?
- Methodological Answer : Use stopped-flow spectrophotometry to capture rapid enzymatic conversions, with protocols adapted from β-hydroxybutyryl-CoA dehydrogenase studies . Fluorescent probes (e.g., thioester-sensitive dyes) enable live-cell imaging. Include technical replicates and validate results with orthogonal methods (e.g., enzymatic vs. LC-MS quantification) .
Q. How should researchers plan multi-omic studies to investigate this compound dynamics?
- Methodological Answer :
Integrate transcriptomics (RNA-seq), proteomics (LC-MS/MS), and metabolomics (GC-/LC-MS) datasets using bioinformatics pipelines like Mimoza for pathway visualization . Power calculations based on pilot data ensure statistical robustness. Pre-register hypotheses and analytical plans to mitigate bias, following open science guidelines .
Propriétés
Formule moléculaire |
C33H58N7O18P3S |
---|---|
Poids moléculaire |
965.8 g/mol |
Nom IUPAC |
S-[2-[3-[[(2R)-4-[[[(2R,3S,4R,5R)-5-(6-aminopurin-9-yl)-4-hydroxy-3-phosphonooxyoxolan-2-yl]methoxy-hydroxyphosphoryl]oxy-hydroxyphosphoryl]oxy-2-hydroxy-3,3-dimethylbutanoyl]amino]propanoylamino]ethyl] (3S)-3-hydroxydodecanethioate |
InChI |
InChI=1S/C33H58N7O18P3S/c1-4-5-6-7-8-9-10-11-21(41)16-24(43)62-15-14-35-23(42)12-13-36-31(46)28(45)33(2,3)18-55-61(52,53)58-60(50,51)54-17-22-27(57-59(47,48)49)26(44)32(56-22)40-20-39-25-29(34)37-19-38-30(25)40/h19-22,26-28,32,41,44-45H,4-18H2,1-3H3,(H,35,42)(H,36,46)(H,50,51)(H,52,53)(H2,34,37,38)(H2,47,48,49)/t21-,22+,26+,27+,28-,32+/m0/s1 |
Clé InChI |
IJFLXRCJWPKGKJ-LXIXEQKWSA-N |
SMILES isomérique |
CCCCCCCCC[C@@H](CC(=O)SCCNC(=O)CCNC(=O)[C@@H](C(C)(C)COP(=O)(O)OP(=O)(O)OC[C@@H]1[C@H]([C@H]([C@@H](O1)N2C=NC3=C(N=CN=C32)N)O)OP(=O)(O)O)O)O |
SMILES canonique |
CCCCCCCCCC(CC(=O)SCCNC(=O)CCNC(=O)C(C(C)(C)COP(=O)(O)OP(=O)(O)OCC1C(C(C(O1)N2C=NC3=C(N=CN=C32)N)O)OP(=O)(O)O)O)O |
Origine du produit |
United States |
Retrosynthesis Analysis
AI-Powered Synthesis Planning: Our tool employs the Template_relevance Pistachio, Template_relevance Bkms_metabolic, Template_relevance Pistachio_ringbreaker, Template_relevance Reaxys, Template_relevance Reaxys_biocatalysis model, leveraging a vast database of chemical reactions to predict feasible synthetic routes.
One-Step Synthesis Focus: Specifically designed for one-step synthesis, it provides concise and direct routes for your target compounds, streamlining the synthesis process.
Accurate Predictions: Utilizing the extensive PISTACHIO, BKMS_METABOLIC, PISTACHIO_RINGBREAKER, REAXYS, REAXYS_BIOCATALYSIS database, our tool offers high-accuracy predictions, reflecting the latest in chemical research and data.
Strategy Settings
Precursor scoring | Relevance Heuristic |
---|---|
Min. plausibility | 0.01 |
Model | Template_relevance |
Template Set | Pistachio/Bkms_metabolic/Pistachio_ringbreaker/Reaxys/Reaxys_biocatalysis |
Top-N result to add to graph | 6 |
Feasible Synthetic Routes
Avertissement et informations sur les produits de recherche in vitro
Veuillez noter que tous les articles et informations sur les produits présentés sur BenchChem sont destinés uniquement à des fins informatives. Les produits disponibles à l'achat sur BenchChem sont spécifiquement conçus pour des études in vitro, qui sont réalisées en dehors des organismes vivants. Les études in vitro, dérivées du terme latin "in verre", impliquent des expériences réalisées dans des environnements de laboratoire contrôlés à l'aide de cellules ou de tissus. Il est important de noter que ces produits ne sont pas classés comme médicaments et n'ont pas reçu l'approbation de la FDA pour la prévention, le traitement ou la guérison de toute condition médicale, affection ou maladie. Nous devons souligner que toute forme d'introduction corporelle de ces produits chez les humains ou les animaux est strictement interdite par la loi. Il est essentiel de respecter ces directives pour assurer la conformité aux normes légales et éthiques en matière de recherche et d'expérimentation.