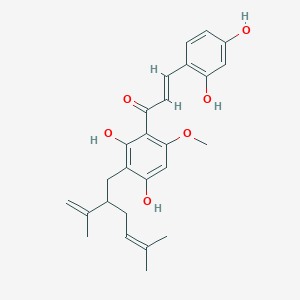
Kuraridine
Vue d'ensemble
Description
Kuraridine is a prenylated flavonol extracted from the roots of Sophora flavescens . It has been identified as a potent inhibitor of cGMP specific phosphodiesterase type 5 (PDE5) .
Synthesis Analysis
Kuraridine is a natural product extracted from the roots of Sophora flavescens . The detailed synthesis process is not available in the retrieved information.Molecular Structure Analysis
The molecular formula of Kuraridine is C26H30O6 . It has a molecular weight of 438.51 . The structure of Kuraridine is classified under Flavonoids, Chalcones, Phenols, and Polyphenols .Chemical Reactions Analysis
Kuraridine shows potent inhibitory activity against cGMP PDE5 . It induces pronounced chloride inward currents in the absence of GABA . The detailed chemical reactions involving Kuraridine are not available in the retrieved information.Physical And Chemical Properties Analysis
Kuraridine is a solid substance with a color ranging from white to yellow . It is soluble in DMSO with a solubility of ≥ 100 mg/mL . .Applications De Recherche Scientifique
Antioxidant Activity
Kuraridine, an isoprenoid flavonoid from Sophora flavescens, has been found to have significant antioxidant activity. In a study, two new compounds, kurarinol A-B, exhibited potent ABTS inhibitory activity, indicating their potential as antioxidants . The antioxidant activity was assessed through ABTS, PTIO, and DPPH methodologies .
Anti-tumor Activity
Kuraridine also demonstrated remarkable cytotoxicity against three cancer cells lines (HepG2, A549, and MCF7) with IC50 values ranging from 7.50–10.55 μM . However, it showed little effect on normal cells . This suggests that kuraridine could be a promising anti-tumor agent .
Mécanisme D'action
Target of Action
Kuraridine, a prenylated flavonol extracted from the roots of Sophora flavescens , primarily targets the cGMP specific phosphodiesterase type 5 (PDE5) . PDE5 is an enzyme that breaks down cGMP, a molecule that plays a crucial role in vasodilation and smooth muscle relaxation .
Mode of Action
Kuraridine exhibits potent inhibitory activity against cGMP PDE5 . By inhibiting PDE5, Kuraridine prevents the breakdown of cGMP, leading to an increase in cGMP levels . This increase in cGMP enhances vasodilation and smooth muscle relaxation . Additionally, Kuraridine has been found to induce pronounced chloride inward currents in the absence of GABA .
Biochemical Pathways
The primary biochemical pathway affected by Kuraridine is the cGMP-PDE5 pathway . By inhibiting PDE5, Kuraridine increases the levels of cGMP, which in turn activates protein kinase G (PKG). PKG then phosphorylates multiple targets, leading to smooth muscle relaxation and vasodilation .
Pharmacokinetics
It’s known that kuraridine is soluble in dmso , which suggests that it may have good bioavailability
Result of Action
The molecular and cellular effects of Kuraridine’s action primarily involve the relaxation of smooth muscles and vasodilation . These effects are due to the increased levels of cGMP resulting from the inhibition of PDE5 . Additionally, Kuraridine has been found to induce chloride inward currents in the absence of GABA , suggesting potential effects on neuronal signaling.
Propriétés
IUPAC Name |
(E)-1-[2,4-dihydroxy-6-methoxy-3-(5-methyl-2-prop-1-en-2-ylhex-4-enyl)phenyl]-3-(2,4-dihydroxyphenyl)prop-2-en-1-one | |
---|---|---|
Source | PubChem | |
URL | https://pubchem.ncbi.nlm.nih.gov | |
Description | Data deposited in or computed by PubChem | |
InChI |
InChI=1S/C26H30O6/c1-15(2)6-7-18(16(3)4)12-20-23(30)14-24(32-5)25(26(20)31)21(28)11-9-17-8-10-19(27)13-22(17)29/h6,8-11,13-14,18,27,29-31H,3,7,12H2,1-2,4-5H3/b11-9+ | |
Source | PubChem | |
URL | https://pubchem.ncbi.nlm.nih.gov | |
Description | Data deposited in or computed by PubChem | |
InChI Key |
PIAPWPAWQGDOMN-PKNBQFBNSA-N | |
Source | PubChem | |
URL | https://pubchem.ncbi.nlm.nih.gov | |
Description | Data deposited in or computed by PubChem | |
Canonical SMILES |
CC(=CCC(CC1=C(C(=C(C=C1O)OC)C(=O)C=CC2=C(C=C(C=C2)O)O)O)C(=C)C)C | |
Source | PubChem | |
URL | https://pubchem.ncbi.nlm.nih.gov | |
Description | Data deposited in or computed by PubChem | |
Isomeric SMILES |
CC(=CCC(CC1=C(C(=C(C=C1O)OC)C(=O)/C=C/C2=C(C=C(C=C2)O)O)O)C(=C)C)C | |
Source | PubChem | |
URL | https://pubchem.ncbi.nlm.nih.gov | |
Description | Data deposited in or computed by PubChem | |
Molecular Formula |
C26H30O6 | |
Source | PubChem | |
URL | https://pubchem.ncbi.nlm.nih.gov | |
Description | Data deposited in or computed by PubChem | |
Molecular Weight |
438.5 g/mol | |
Source | PubChem | |
URL | https://pubchem.ncbi.nlm.nih.gov | |
Description | Data deposited in or computed by PubChem | |
Product Name |
Kuraridin |
Retrosynthesis Analysis
AI-Powered Synthesis Planning: Our tool employs the Template_relevance Pistachio, Template_relevance Bkms_metabolic, Template_relevance Pistachio_ringbreaker, Template_relevance Reaxys, Template_relevance Reaxys_biocatalysis model, leveraging a vast database of chemical reactions to predict feasible synthetic routes.
One-Step Synthesis Focus: Specifically designed for one-step synthesis, it provides concise and direct routes for your target compounds, streamlining the synthesis process.
Accurate Predictions: Utilizing the extensive PISTACHIO, BKMS_METABOLIC, PISTACHIO_RINGBREAKER, REAXYS, REAXYS_BIOCATALYSIS database, our tool offers high-accuracy predictions, reflecting the latest in chemical research and data.
Strategy Settings
Precursor scoring | Relevance Heuristic |
---|---|
Min. plausibility | 0.01 |
Model | Template_relevance |
Template Set | Pistachio/Bkms_metabolic/Pistachio_ringbreaker/Reaxys/Reaxys_biocatalysis |
Top-N result to add to graph | 6 |
Feasible Synthetic Routes
Q & A
Q1: What is Kuraridine and what is its known biological activity?
A1: Kuraridine is a lavandulylated chalcone found in the Chinese crude drug Sophora flavescens, also known as Ku Shen. [, ] It exhibits antimicrobial activity against Staphylococcus aureus and Streptococcus mutans. [] Interestingly, it is also the first reported chalcone to show glycosidase inhibitory activity, specifically against beta-glucosidase with an IC50 value of 57 μM. []
Q2: How potent is Kuraridine as a glycosidase inhibitor compared to other compounds found in Sophora flavescens?
A2: While Kuraridine shows moderate activity against beta-glucosidase, other lavandulylated flavonoids isolated from Sophora flavescens, such as kushenol A, (-)-kurarinone, and sophoraflavanone G, exhibit stronger inhibitory activities against alpha-glucosidase. [, ] This suggests that the specific structure and substitutions on the flavanone backbone might be crucial for enhancing activity against specific glycosidase enzymes.
Q3: Does the presence of a lavandulyl group influence the biological activity of Kuraridine?
A3: Research suggests that the 8-lavandulyl group in the B-ring of flavonoids significantly contributes to glycosidase inhibitory activities. [] Although Kuraridine is a chalcone and not a flavonoid, it also possesses a lavandulyl group. Further studies are needed to understand the specific role of the lavandulyl group in Kuraridine's interaction with beta-glucosidase.
Q4: Are there any studies investigating the structure-activity relationship of Kuraridine and its analogs?
A4: While the provided research highlights the importance of the lavandulyl group for glycosidase inhibition, [] specific studies focusing on the structure-activity relationship of Kuraridine and its analogs are currently lacking. Investigating how modifications to the Kuraridine scaffold affect its activity, potency, and selectivity towards different glycosidases could be valuable for future drug development.
Avertissement et informations sur les produits de recherche in vitro
Veuillez noter que tous les articles et informations sur les produits présentés sur BenchChem sont destinés uniquement à des fins informatives. Les produits disponibles à l'achat sur BenchChem sont spécifiquement conçus pour des études in vitro, qui sont réalisées en dehors des organismes vivants. Les études in vitro, dérivées du terme latin "in verre", impliquent des expériences réalisées dans des environnements de laboratoire contrôlés à l'aide de cellules ou de tissus. Il est important de noter que ces produits ne sont pas classés comme médicaments et n'ont pas reçu l'approbation de la FDA pour la prévention, le traitement ou la guérison de toute condition médicale, affection ou maladie. Nous devons souligner que toute forme d'introduction corporelle de ces produits chez les humains ou les animaux est strictement interdite par la loi. Il est essentiel de respecter ces directives pour assurer la conformité aux normes légales et éthiques en matière de recherche et d'expérimentation.