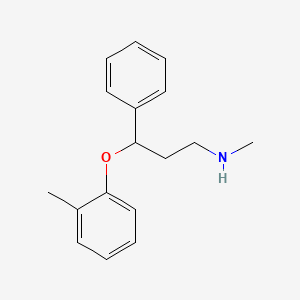
Atomoxétine
Vue d'ensemble
Description
La Tomoxétine, également connue sous le nom d'Atomoxetine, est un inhibiteur sélectif de la recapture de la norépinéphrine (IRSN) principalement utilisé dans le traitement du trouble déficitaire de l'attention avec hyperactivité (TDAH). Elle est commercialisée sous le nom de marque Strattera. Contrairement aux médicaments stimulants traditionnels pour le TDAH, la Tomoxétine est un non-stimulant, ce qui en fait une option unique pour les patients qui peuvent ne pas bien répondre aux stimulants ou qui ont des contre-indications à leur utilisation .
Applications De Recherche Scientifique
Tomoxetine has a wide range of scientific research applications:
Chemistry: Used as a model compound to study selective norepinephrine reuptake inhibition.
Biology: Investigated for its effects on neurotransmitter systems, particularly norepinephrine and dopamine.
Industry: Utilized in the development of non-stimulant medications for ADHD and related disorders.
Mécanisme D'action
Target of Action
Atomoxetine, also known as Tomoxetine, primarily targets the norepinephrine transporter (NET) . This transporter is responsible for the reuptake of norepinephrine (NE) in the brain. By inhibiting NET, Atomoxetine increases the availability of NE in the synaptic cleft . Additionally, Atomoxetine has been shown to inhibit the reuptake of dopamine in specific brain regions such as the prefrontal cortex (PFC) .
Mode of Action
Atomoxetine acts as a selective norepinephrine reuptake inhibitor (SNRI) . It binds to NET, preventing the reuptake of NE throughout the brain . This increases the concentration of NE in the synaptic cleft, enhancing noradrenergic transmission . This selective action is beneficial in the treatment of ADHD, as it avoids many of the side effects associated with stimulant medications .
Biochemical Pathways
The increased levels of NE and dopamine in the brain, particularly in the PFC, improve symptoms associated with ADHD, including distractibility, short attention span, hyperactivity, emotional lability, and impulsivity . Dysregulation in noradrenergic and dopaminergic pathways is thought to play a critical role in suboptimal executive functioning within prefrontal regions of the brain, which are involved in attention and memory .
Pharmacokinetics
Atomoxetine is rapidly and completely absorbed after oral administration, with an absolute oral bioavailability ranging from 63 to 94% . It is primarily metabolized by the highly polymorphic drug metabolizing enzyme cytochrome P450 2D6 (CYP2D6) . The systemic plasma clearance of atomoxetine is 0.35 L/h/kg in extensive metabolizers and 0.03 L/h/kg in poor metabolizers . The average steady-state plasma concentrations are approximately 10-fold higher in poor metabolizers compared with extensive metabolizers .
Result of Action
The inhibition of NE reuptake by Atomoxetine leads to clinically meaningful improvements in the core symptoms of ADHD (inattention, impulsivity, and hyperactivity) as well as quality of life and emotional lability . It is effective and generally well tolerated .
Action Environment
Atomoxetine’s action can be influenced by environmental factors such as the presence of other medications. For instance, its noradrenergic activity can lead to effects on the cardiovascular system such as increased blood pressure and tachycardia . Therefore, Atomoxetine should be used with caution in patients with underlying medical conditions .
Analyse Biochimique
Biochemical Properties
Tomoxetine plays a significant role in biochemical reactions by inhibiting the norepinephrine transporter (NET), which prevents the reuptake of norepinephrine into presynaptic neurons. This inhibition leads to an increase in the concentration of norepinephrine in the synaptic cleft, enhancing neurotransmission. Tomoxetine also interacts with other biomolecules, including the serotonin transporter (SERT) and the N-methyl-D-aspartate (NMDA) receptor . These interactions contribute to its therapeutic effects and influence various biochemical pathways.
Cellular Effects
Tomoxetine affects various types of cells and cellular processes. It influences cell function by modulating cell signaling pathways, gene expression, and cellular metabolism. In particular, Tomoxetine increases the levels of norepinephrine and dopamine in the prefrontal cortex, which is associated with improved attention and executive function . Additionally, Tomoxetine’s interaction with the NMDA receptor suggests a role in modulating glutamatergic neurotransmission, which may impact synaptic plasticity and cognitive function .
Molecular Mechanism
The molecular mechanism of Tomoxetine involves its binding to the norepinephrine transporter (NET), inhibiting the reuptake of norepinephrine into presynaptic neurons. This inhibition increases the availability of norepinephrine in the synaptic cleft, enhancing adrenergic signaling. Tomoxetine also binds to the serotonin transporter (SERT) and blocks the NMDA receptor, indicating its involvement in serotonergic and glutamatergic neurotransmission . These interactions contribute to its therapeutic effects in managing ADHD symptoms.
Temporal Effects in Laboratory Settings
In laboratory settings, the effects of Tomoxetine change over time. Studies have shown that initial responses to Tomoxetine may be apparent within one week of treatment, but robust responses often build gradually over several weeks . The stability and degradation of Tomoxetine in laboratory settings are influenced by its metabolic pathways, primarily involving cytochrome P450 (CYP) 2D6 . Long-term effects on cellular function have been observed, with sustained improvements in attention and executive function over extended treatment periods .
Dosage Effects in Animal Models
The effects of Tomoxetine vary with different dosages in animal models. In studies using spontaneously hypertensive rats (SHR), a model for ADHD, Tomoxetine at a dose of 1 mg/kg/day showed significant improvements in motor activity compared to lower doses . Higher doses of Tomoxetine have been associated with dose-dependent decreases in dopamine D2 receptor expression in specific brain regions, such as the prefrontal cortex, striatum, and hypothalamus . High doses may also lead to adverse effects, including increased risk of cardiovascular events .
Metabolic Pathways
Tomoxetine is primarily metabolized by cytochrome P450 (CYP) 2D6, which catalyzes its aromatic ring-hydroxylation, benzylic hydroxylation, and N-demethylation . The primary oxidative metabolite, 4-hydroxyatomoxetine, is subsequently glucuronidated and excreted in urine . The activity of CYP2D6 varies among individuals, leading to differences in Tomoxetine’s pharmacokinetics and therapeutic response . Additionally, other enzymes, such as CYP2E1 and CYP3A, contribute to its metabolism in individuals with lower CYP2D6 activity .
Transport and Distribution
Tomoxetine is rapidly absorbed after oral administration, with peak plasma concentrations occurring within 1-2 hours . It has a high volume of distribution, indicating extensive distribution in body tissues . Tomoxetine is highly bound to plasma proteins, primarily albumin, which affects its transport and distribution within cells and tissues . The steady-state volume of distribution is approximately 0.85 L/kg .
Subcellular Localization
The subcellular localization of Tomoxetine is influenced by its interactions with specific transporters and binding proteins. Tomoxetine’s binding to the norepinephrine transporter (NET) and serotonin transporter (SERT) suggests its localization at synaptic terminals where these transporters are expressed . Additionally, its interaction with the NMDA receptor indicates potential localization at postsynaptic sites involved in glutamatergic neurotransmission . These interactions and localizations contribute to Tomoxetine’s therapeutic effects on neurotransmission and cognitive function.
Méthodes De Préparation
Voies de synthèse et conditions de réaction
La Tomoxétine peut être synthétisée par plusieurs méthodes. Une voie de synthèse courante implique la réaction de la N-méthyl-3-hydroxy-3-phénylpropylamine avec le 2-fluorotoluène en présence d'une base. Cette réaction forme la Tomoxétine comme produit principal . Une autre méthode consiste à chauffer un alcali et de la N-méthyl-3-hydroxy-3-phénylpropylamine pour former un mélange, suivi de l'ajout de 2-fluorotoluène .
Méthodes de production industrielle
La production industrielle de Tomoxétine implique généralement une synthèse à grande échelle utilisant les méthodes mentionnées ci-dessus. Le processus est optimisé pour un rendement et une pureté élevés, garantissant que le produit final répond aux normes pharmaceutiques. Les conditions de réaction sont soigneusement contrôlées pour minimiser les impuretés et maximiser l'efficacité de la synthèse .
Analyse Des Réactions Chimiques
Types de réactions
La Tomoxétine subit plusieurs types de réactions chimiques, notamment :
Oxydation : Le principal métabolite oxydatif formé est la 4-hydroxy-Tomoxétine, qui est rapidement glucuronidée.
Réactifs et conditions courants
Oxydation : Les réactifs courants comprennent des agents oxydants comme le peroxyde d'hydrogène ou le permanganate de potassium.
Substitution : Les conditions impliquent généralement l'utilisation de solvants comme l'acétonitrile et de réactifs tels que le p-chloranil et le DDQ.
Principaux produits formés
Oxydation : Le produit principal est la 4-hydroxy-Tomoxétine.
Substitution : Les produits principaux sont des complexes de transfert de charge avec des accepteurs π à base de quinone.
Applications de recherche scientifique
La Tomoxétine a un large éventail d'applications de recherche scientifique :
Chimie : Utilisée comme composé modèle pour étudier l'inhibition sélective de la recapture de la norépinéphrine.
Biologie : Étudiée pour ses effets sur les systèmes de neurotransmetteurs, en particulier la norépinéphrine et la dopamine.
Industrie : Utilisée dans le développement de médicaments non stimulants pour le TDAH et les troubles connexes.
Mécanisme d'action
La Tomoxétine exerce ses effets en inhibant sélectivement le transporteur présynaptique de la norépinéphrine (NET), empêchant la recapture de la norépinéphrine dans tout le cerveau. Cela augmente les niveaux extracellulaires de norépinéphrine et de dopamine dans des régions cérébrales spécifiques, telles que le cortex préfrontal, qui sont impliquées dans l'attention et la mémoire . De plus, il a été démontré que la Tomoxétine se lie au transporteur de la sérotonine (SERT) et bloque le récepteur N-méthyl-D-aspartate (NMDA), indiquant un rôle pour le système glutamatergique dans son mécanisme d'action .
Comparaison Avec Des Composés Similaires
Composés similaires
Méthylphénidate : Un médicament stimulant couramment utilisé pour traiter le TDAH. Contrairement à la Tomoxétine, il augmente principalement les niveaux de dopamine dans le cerveau.
Dextroamphétamine : Un autre stimulant utilisé pour le TDAH, qui augmente également les niveaux de dopamine et de norépinéphrine.
Guanfacine : Un médicament non stimulant qui agit sur les récepteurs alpha-2 adrénergiques, utilisé pour le traitement du TDAH.
Unicité de la Tomoxétine
La Tomoxétine est unique parmi les médicaments contre le TDAH en raison de sa nature non stimulante et de son inhibition sélective de la recapture de la norépinéphrine. Cela en fait une option précieuse pour les patients qui peuvent ne pas tolérer les médicaments stimulants ou qui ont des contre-indications à leur utilisation .
Propriétés
IUPAC Name |
N-methyl-3-(2-methylphenoxy)-3-phenylpropan-1-amine | |
---|---|---|
Source | PubChem | |
URL | https://pubchem.ncbi.nlm.nih.gov | |
Description | Data deposited in or computed by PubChem | |
InChI |
InChI=1S/C17H21NO/c1-14-8-6-7-11-16(14)19-17(12-13-18-2)15-9-4-3-5-10-15/h3-11,17-18H,12-13H2,1-2H3 | |
Source | PubChem | |
URL | https://pubchem.ncbi.nlm.nih.gov | |
Description | Data deposited in or computed by PubChem | |
InChI Key |
VHGCDTVCOLNTBX-UHFFFAOYSA-N | |
Source | PubChem | |
URL | https://pubchem.ncbi.nlm.nih.gov | |
Description | Data deposited in or computed by PubChem | |
Canonical SMILES |
CC1=CC=CC=C1OC(CCNC)C2=CC=CC=C2 | |
Source | PubChem | |
URL | https://pubchem.ncbi.nlm.nih.gov | |
Description | Data deposited in or computed by PubChem | |
Molecular Formula |
C17H21NO | |
Source | PubChem | |
URL | https://pubchem.ncbi.nlm.nih.gov | |
Description | Data deposited in or computed by PubChem | |
DSSTOX Substance ID |
DTXSID10861048 | |
Record name | N-Methyl-3-(2-methylphenoxy)-3-phenyl-1-propanamine | |
Source | EPA DSSTox | |
URL | https://comptox.epa.gov/dashboard/DTXSID10861048 | |
Description | DSSTox provides a high quality public chemistry resource for supporting improved predictive toxicology. | |
Molecular Weight |
255.35 g/mol | |
Source | PubChem | |
URL | https://pubchem.ncbi.nlm.nih.gov | |
Description | Data deposited in or computed by PubChem | |
CAS No. |
63940-51-2 | |
Record name | N-Methyl-γ-(2-methylphenoxy)benzenepropanamine | |
Source | CAS Common Chemistry | |
URL | https://commonchemistry.cas.org/detail?cas_rn=63940-51-2 | |
Description | CAS Common Chemistry is an open community resource for accessing chemical information. Nearly 500,000 chemical substances from CAS REGISTRY cover areas of community interest, including common and frequently regulated chemicals, and those relevant to high school and undergraduate chemistry classes. This chemical information, curated by our expert scientists, is provided in alignment with our mission as a division of the American Chemical Society. | |
Explanation | The data from CAS Common Chemistry is provided under a CC-BY-NC 4.0 license, unless otherwise stated. | |
Record name | N-Methyl-3-(2-methylphenoxy)-3-phenyl-1-propanamine | |
Source | EPA DSSTox | |
URL | https://comptox.epa.gov/dashboard/DTXSID10861048 | |
Description | DSSTox provides a high quality public chemistry resource for supporting improved predictive toxicology. | |
Record name | N-methyl-3-(2-methylphenoxy)-3-phenylpropan-1-amine | |
Source | European Chemicals Agency (ECHA) | |
URL | https://echa.europa.eu/substance-information/-/substanceinfo/100.219.386 | |
Description | The European Chemicals Agency (ECHA) is an agency of the European Union which is the driving force among regulatory authorities in implementing the EU's groundbreaking chemicals legislation for the benefit of human health and the environment as well as for innovation and competitiveness. | |
Explanation | Use of the information, documents and data from the ECHA website is subject to the terms and conditions of this Legal Notice, and subject to other binding limitations provided for under applicable law, the information, documents and data made available on the ECHA website may be reproduced, distributed and/or used, totally or in part, for non-commercial purposes provided that ECHA is acknowledged as the source: "Source: European Chemicals Agency, http://echa.europa.eu/". Such acknowledgement must be included in each copy of the material. ECHA permits and encourages organisations and individuals to create links to the ECHA website under the following cumulative conditions: Links can only be made to webpages that provide a link to the Legal Notice page. | |
Synthesis routes and methods
Procedure details
Retrosynthesis Analysis
AI-Powered Synthesis Planning: Our tool employs the Template_relevance Pistachio, Template_relevance Bkms_metabolic, Template_relevance Pistachio_ringbreaker, Template_relevance Reaxys, Template_relevance Reaxys_biocatalysis model, leveraging a vast database of chemical reactions to predict feasible synthetic routes.
One-Step Synthesis Focus: Specifically designed for one-step synthesis, it provides concise and direct routes for your target compounds, streamlining the synthesis process.
Accurate Predictions: Utilizing the extensive PISTACHIO, BKMS_METABOLIC, PISTACHIO_RINGBREAKER, REAXYS, REAXYS_BIOCATALYSIS database, our tool offers high-accuracy predictions, reflecting the latest in chemical research and data.
Strategy Settings
Precursor scoring | Relevance Heuristic |
---|---|
Min. plausibility | 0.01 |
Model | Template_relevance |
Template Set | Pistachio/Bkms_metabolic/Pistachio_ringbreaker/Reaxys/Reaxys_biocatalysis |
Top-N result to add to graph | 6 |
Feasible Synthetic Routes
Q1: What is the primary mechanism of action of tomoxetine?
A1: Tomoxetine is a potent and selective inhibitor of norepinephrine (NE) reuptake. [] It exerts its therapeutic effects by increasing the concentration of NE in synaptic clefts, primarily in the prefrontal cortex, an area of the brain associated with attention, executive function, and working memory. [, , , , ]
Q2: How does tomoxetine's mechanism of action differ from that of psychostimulants like methylphenidate?
A2: Unlike psychostimulants, which primarily target dopamine transporters in addition to NE transporters, tomoxetine exhibits selectivity for NE transporters. [, , , ] This selectivity may translate to a different side effect profile, potentially reducing the risk of certain adverse effects associated with dopamine modulation. [, ]
Q3: Does tomoxetine have any effect on dopamine levels in the brain?
A3: While tomoxetine primarily targets NE reuptake, studies in animals suggest that it can indirectly increase dopamine levels in the cortex without significantly affecting subcortical dopamine levels. [] This effect on cortical dopamine is thought to contribute to its therapeutic benefits in ADHD. []
Q4: What is the molecular formula and weight of tomoxetine?
A4: The molecular formula of tomoxetine is C17H21NO • HCl (tomoxetine hydrochloride). Its molecular weight is 291.82 g/mol.
Q5: How does the structure of tomoxetine contribute to its selectivity for norepinephrine transporters over serotonin transporters?
A5: The p-trifluoromethyl substituent on the phenoxy ring of tomoxetine plays a crucial role in its selectivity for NE transporters. [] Analogs lacking this substituent or with modifications at this position exhibit significantly reduced potency and selectivity for NE uptake inhibition. [, ]
Q6: Have any structural modifications of tomoxetine been explored, and how do they affect its activity?
A6: Research has explored modifications to the tomoxetine structure, primarily by altering the substituents on the phenoxy ring. [] Replacing the p-trifluoromethyl group with other substituents, such as iodine or methoxy groups, has been shown to alter the compound's selectivity for monoamine transporters. [] For instance, iodinated derivatives displayed high affinity for serotonin transporters, while retaining some affinity for NE transporters. []
Q7: What is the bioavailability of tomoxetine after oral administration?
A7: Oral bioavailability of tomoxetine varies significantly between species. Studies in rats revealed low oral bioavailability (F = 4%), attributed to efficient first-pass hepatic metabolism. [] In contrast, dogs exhibited high oral bioavailability (F = 74%), indicating reduced first-pass metabolism in this species. []
Q8: How is tomoxetine metabolized, and what are the major routes of elimination?
A8: Tomoxetine undergoes extensive metabolism, primarily in the liver. [] Major metabolic pathways include aromatic ring hydroxylation, benzylic oxidation (observed in rats), and N-demethylation. [] The primary metabolite, 4-hydroxytomoxetine, is further conjugated to form glucuronide and sulfate conjugates (observed in dogs). [] Excretion occurs mainly through urine (48-66% of the dose) and feces (32-42% of the dose) in both rats and dogs. []
Q9: Does tomoxetine exhibit dose-dependent pharmacokinetics?
A9: Studies in humans suggest potential dose-dependent pharmacokinetics of tomoxetine. [] While a majority of subjects displayed a typical half-life of approximately 4-5 hours after single or multiple doses, some individuals exhibited significantly longer half-lives (around 19 hours), suggesting potential for accumulation in a subset of the population. []
Q10: What preclinical models have been used to evaluate the efficacy of tomoxetine in attention-deficit/hyperactivity disorder (ADHD)?
A10: Preclinical studies have employed various animal models to assess tomoxetine's potential in ADHD. Rodent models of impulsivity, attention, and hyperactivity are frequently utilized. [, ] Additionally, research has explored the effects of tomoxetine on neurochemical markers and electrophysiological activity in brain regions implicated in ADHD pathology. [, , , ]
Q11: Has tomoxetine demonstrated efficacy in clinical trials for ADHD?
A11: Yes, clinical trials have demonstrated the efficacy of tomoxetine in treating ADHD in both children and adults. [, , , , ] Significant improvements in core ADHD symptoms, including inattention, hyperactivity, and impulsivity, have been observed compared to placebo. [, , , , ]
Q12: What analytical methods are commonly employed for the detection and quantification of tomoxetine in biological samples?
A13: Several analytical methods have been developed and validated for the determination of tomoxetine in various matrices. Liquid chromatography coupled with mass spectrometry (LC-MS) is widely used for its sensitivity and selectivity in quantifying tomoxetine and its metabolites in plasma and other biological fluids. [] Gas chromatography, particularly headspace gas chromatography (HS-GC), has been employed to quantify residual organic solvents in tomoxetine hydrochloride drug substance. []
Q13: Does tomoxetine interact with any drug transporters other than norepinephrine transporters?
A14: While tomoxetine exhibits high selectivity for NE transporters, in vitro studies using rat brain tissue suggest that it can also bind to serotonin transporters, although with lower affinity compared to NE transporters. [] This finding highlights the importance of further investigating potential drug-transporter interactions, particularly at higher doses or in individuals with specific genetic variations in transporter expression or function.
Q14: What are the main alternatives to tomoxetine for treating ADHD, and how do their mechanisms of action compare?
A15: The primary alternatives to tomoxetine for ADHD treatment are psychostimulants, such as methylphenidate and amphetamine derivatives. [] These medications primarily act by blocking both dopamine and NE transporters, leading to increased levels of these neurotransmitters in the synaptic cleft. [] Unlike tomoxetine, which selectively targets NE reuptake, psychostimulants have a more pronounced effect on dopamine levels, particularly in the mesolimbic pathway, which is associated with reward and motivation. []
Q15: What were the initial therapeutic targets of tomoxetine before its development for ADHD?
A16: Tomoxetine was initially investigated in the 1980s as a potential treatment for depressive illness due to its selective inhibition of norepinephrine reuptake. [, ] While early clinical trials demonstrated some efficacy in treating depression, its development for this indication was discontinued. [, ] It was later in the 1990s that research refocused on its potential in treating ADHD, leading to its approval for this indication. []
Avertissement et informations sur les produits de recherche in vitro
Veuillez noter que tous les articles et informations sur les produits présentés sur BenchChem sont destinés uniquement à des fins informatives. Les produits disponibles à l'achat sur BenchChem sont spécifiquement conçus pour des études in vitro, qui sont réalisées en dehors des organismes vivants. Les études in vitro, dérivées du terme latin "in verre", impliquent des expériences réalisées dans des environnements de laboratoire contrôlés à l'aide de cellules ou de tissus. Il est important de noter que ces produits ne sont pas classés comme médicaments et n'ont pas reçu l'approbation de la FDA pour la prévention, le traitement ou la guérison de toute condition médicale, affection ou maladie. Nous devons souligner que toute forme d'introduction corporelle de ces produits chez les humains ou les animaux est strictement interdite par la loi. Il est essentiel de respecter ces directives pour assurer la conformité aux normes légales et éthiques en matière de recherche et d'expérimentation.