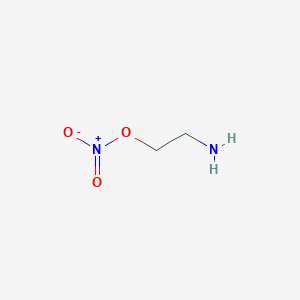
Nitrate d'aminoéthyle
Vue d'ensemble
Description
Applications De Recherche Scientifique
Aminoethyl nitrate has several scientific research applications, including:
Mécanisme D'action
Target of Action
Aminoethyl nitrate, also known as 2-Aminoethyl nitrate, is a potent vasodilator . The primary targets of this compound are the blood vessels, specifically the smooth muscle cells that line the vessels . These cells play a crucial role in controlling vascular tone and blood pressure .
Mode of Action
Aminoethyl nitrate functions as a source of nitric oxide . Nitric oxide is a potent signaling molecule that induces relaxation of the involuntary muscles, including the smooth muscle cells of the blood vessels . This relaxation leads to vasodilation, or the widening of the blood vessels, which in turn results in a decrease in blood pressure .
Biochemical Pathways
The action of Aminoethyl nitrate affects several biochemical pathways. Nitric oxide, produced by Aminoethyl nitrate, is involved in various nitrogen metabolism pathways, including nitrogen fixation, nitrification, denitrification, dissimilatory nitrate reduction, assimilatory nitrate reduction, and complete nitrification . These pathways have downstream effects on various biological processes, including the regulation of blood pressure and the immune response .
Pharmacokinetics
It is known that similar compounds, such as amyl nitrite, are rapidly absorbed through the pulmonary alveoli, manifesting therapeutic effects within one minute after inhalation
Result of Action
The primary result of Aminoethyl nitrate’s action is a decrease in blood pressure due to the dilation of blood vessels . This can provide rapid relief for conditions such as angina pectoris . It’s important to note that aminoethyl nitrate has been identified as a novel organic mononitrate with high potency but devoid of induction of mitochondrial oxidative stress .
Action Environment
The action, efficacy, and stability of Aminoethyl nitrate can be influenced by various environmental factors. For instance, changes in light, temperature, mineral nutrients, or stress conditions, such as drought, flooding, and salinity, can require metabolic adjustments
Orientations Futures
While there is no conclusive epidemiological evidence to date supporting dietary nitrate intake reducing blood pressure, a number of studies have shown that increased consumption of fruits and vegetables reduces systolic and diastolic blood pressure . This suggests that Aminoethyl nitrate and other nitrates could potentially be used in dietary interventions to improve cardiovascular health.
Analyse Biochimique
Biochemical Properties
Aminoethyl nitrate is a potent vasodilator, similar to other alkyl nitrates . It functions as a source of nitric oxide, which signals for relaxation of the involuntary muscles .
Cellular Effects
Aminoethyl nitrate, like other alkyl nitrates, can cause vasodilation by generating nitric oxide . This can influence cell function by causing relaxation of involuntary muscles, especially the blood vessel walls
Molecular Mechanism
The molecular mechanism of Aminoethyl nitrate is thought to involve the reduction of systemic and pulmonary arterial pressure (afterload) and decreased cardiac output because of peripheral vasodilation . It is a source of nitric oxide, which accounts for the mechanism described above .
Temporal Effects in Laboratory Settings
One study suggests that Aminoethyl nitrate showed an almost similar potency to glyceryl trinitrate (GTN), even though it is only a mononitrate .
Metabolic Pathways
Aminoethyl nitrate is likely involved in the nitrate-nitrite-NO pathway This pathway involves the reduction of nitrate to nitrite and then to nitric oxide
Méthodes De Préparation
Synthetic Routes and Reaction Conditions
Aminoethyl nitrate can be synthesized through the nitration of ethanolamine. The process involves the reaction of ethanolamine with nitric acid, typically in the presence of acetic anhydride as a catalyst . The reaction conditions must be carefully controlled to avoid the formation of unwanted by-products and to ensure the safety of the process, as low molecular weight nitro esters can be highly explosive .
Industrial Production Methods
Industrial production of aminoethyl nitrate follows similar synthetic routes but on a larger scale. The process involves the careful handling of nitric acid and ethanolamine, with stringent safety measures in place to prevent accidents due to the explosive nature of the compound .
Analyse Des Réactions Chimiques
Types of Reactions
Aminoethyl nitrate undergoes several types of chemical reactions, including:
Oxidation: Aminoethyl nitrate can be oxidized to form various nitrogen oxides.
Reduction: The compound can be reduced to form ethanolamine and other nitrogen-containing compounds.
Substitution: Aminoethyl nitrate can participate in substitution reactions where the nitrate group is replaced by other functional groups.
Common Reagents and Conditions
Oxidation: Common oxidizing agents include potassium permanganate and hydrogen peroxide.
Reduction: Reducing agents such as lithium aluminum hydride and hydrogen gas in the presence of a metal catalyst are commonly used.
Substitution: Substitution reactions often involve nucleophiles such as amines or thiols.
Major Products Formed
Oxidation: Nitrogen oxides and other oxidized nitrogen compounds.
Reduction: Ethanolamine and other reduced nitrogen compounds.
Substitution: Various substituted aminoethyl derivatives.
Comparaison Avec Des Composés Similaires
Similar Compounds
Glyceryl trinitrate: Another organic nitrate used for its vasodilatory properties.
Amyl nitrite: A fast-acting vasodilator used for the rapid relief of angina pectoris.
Isosorbide dinitrate: A nitrate used to prevent and treat angina pectoris.
Uniqueness of Aminoethyl Nitrate
Aminoethyl nitrate is unique in that it does not induce mitochondrial oxidative stress or cross-tolerance to other nitrates, such as glyceryl trinitrate . This makes it a promising candidate for long-term therapeutic use without the common side effects associated with other nitrates .
Propriétés
IUPAC Name |
2-aminoethyl nitrate | |
---|---|---|
Source | PubChem | |
URL | https://pubchem.ncbi.nlm.nih.gov | |
Description | Data deposited in or computed by PubChem | |
InChI |
InChI=1S/C2H6N2O3/c3-1-2-7-4(5)6/h1-3H2 | |
Source | PubChem | |
URL | https://pubchem.ncbi.nlm.nih.gov | |
Description | Data deposited in or computed by PubChem | |
InChI Key |
KZTZJUQNSSLNAG-UHFFFAOYSA-N | |
Source | PubChem | |
URL | https://pubchem.ncbi.nlm.nih.gov | |
Description | Data deposited in or computed by PubChem | |
Canonical SMILES |
C(CO[N+](=O)[O-])N | |
Source | PubChem | |
URL | https://pubchem.ncbi.nlm.nih.gov | |
Description | Data deposited in or computed by PubChem | |
Molecular Formula |
C2H6N2O3 | |
Source | PubChem | |
URL | https://pubchem.ncbi.nlm.nih.gov | |
Description | Data deposited in or computed by PubChem | |
DSSTOX Substance ID |
DTXSID40214846 | |
Record name | Aminoethyl nitrate [INN:DCF] | |
Source | EPA DSSTox | |
URL | https://comptox.epa.gov/dashboard/DTXSID40214846 | |
Description | DSSTox provides a high quality public chemistry resource for supporting improved predictive toxicology. | |
Molecular Weight |
106.08 g/mol | |
Source | PubChem | |
URL | https://pubchem.ncbi.nlm.nih.gov | |
Description | Data deposited in or computed by PubChem | |
CAS No. |
646-02-6 | |
Record name | Aminoethyl nitrate [INN:DCF] | |
Source | ChemIDplus | |
URL | https://pubchem.ncbi.nlm.nih.gov/substance/?source=chemidplus&sourceid=0000646026 | |
Description | ChemIDplus is a free, web search system that provides access to the structure and nomenclature authority files used for the identification of chemical substances cited in National Library of Medicine (NLM) databases, including the TOXNET system. | |
Record name | Aminoethyl nitrate | |
Source | DrugBank | |
URL | https://www.drugbank.ca/drugs/DB13585 | |
Description | The DrugBank database is a unique bioinformatics and cheminformatics resource that combines detailed drug (i.e. chemical, pharmacological and pharmaceutical) data with comprehensive drug target (i.e. sequence, structure, and pathway) information. | |
Explanation | Creative Common's Attribution-NonCommercial 4.0 International License (http://creativecommons.org/licenses/by-nc/4.0/legalcode) | |
Record name | Aminoethyl nitrate [INN:DCF] | |
Source | EPA DSSTox | |
URL | https://comptox.epa.gov/dashboard/DTXSID40214846 | |
Description | DSSTox provides a high quality public chemistry resource for supporting improved predictive toxicology. | |
Record name | AMINOETHYL NITRATE | |
Source | FDA Global Substance Registration System (GSRS) | |
URL | https://gsrs.ncats.nih.gov/ginas/app/beta/substances/S1IA7R2J48 | |
Description | The FDA Global Substance Registration System (GSRS) enables the efficient and accurate exchange of information on what substances are in regulated products. Instead of relying on names, which vary across regulatory domains, countries, and regions, the GSRS knowledge base makes it possible for substances to be defined by standardized, scientific descriptions. | |
Explanation | Unless otherwise noted, the contents of the FDA website (www.fda.gov), both text and graphics, are not copyrighted. They are in the public domain and may be republished, reprinted and otherwise used freely by anyone without the need to obtain permission from FDA. Credit to the U.S. Food and Drug Administration as the source is appreciated but not required. | |
Retrosynthesis Analysis
AI-Powered Synthesis Planning: Our tool employs the Template_relevance Pistachio, Template_relevance Bkms_metabolic, Template_relevance Pistachio_ringbreaker, Template_relevance Reaxys, Template_relevance Reaxys_biocatalysis model, leveraging a vast database of chemical reactions to predict feasible synthetic routes.
One-Step Synthesis Focus: Specifically designed for one-step synthesis, it provides concise and direct routes for your target compounds, streamlining the synthesis process.
Accurate Predictions: Utilizing the extensive PISTACHIO, BKMS_METABOLIC, PISTACHIO_RINGBREAKER, REAXYS, REAXYS_BIOCATALYSIS database, our tool offers high-accuracy predictions, reflecting the latest in chemical research and data.
Strategy Settings
Precursor scoring | Relevance Heuristic |
---|---|
Min. plausibility | 0.01 |
Model | Template_relevance |
Template Set | Pistachio/Bkms_metabolic/Pistachio_ringbreaker/Reaxys/Reaxys_biocatalysis |
Top-N result to add to graph | 6 |
Feasible Synthetic Routes
Avertissement et informations sur les produits de recherche in vitro
Veuillez noter que tous les articles et informations sur les produits présentés sur BenchChem sont destinés uniquement à des fins informatives. Les produits disponibles à l'achat sur BenchChem sont spécifiquement conçus pour des études in vitro, qui sont réalisées en dehors des organismes vivants. Les études in vitro, dérivées du terme latin "in verre", impliquent des expériences réalisées dans des environnements de laboratoire contrôlés à l'aide de cellules ou de tissus. Il est important de noter que ces produits ne sont pas classés comme médicaments et n'ont pas reçu l'approbation de la FDA pour la prévention, le traitement ou la guérison de toute condition médicale, affection ou maladie. Nous devons souligner que toute forme d'introduction corporelle de ces produits chez les humains ou les animaux est strictement interdite par la loi. Il est essentiel de respecter ces directives pour assurer la conformité aux normes légales et éthiques en matière de recherche et d'expérimentation.