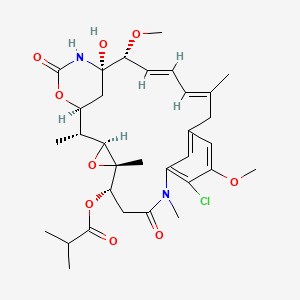
Ansamitocin P-3
Vue d'ensemble
Description
Maytansinolisobutyrate is a natural product found in Actinosynnema pretiosum with data available.
Applications De Recherche Scientifique
Agent anticancéreux
L'AP-3 est un agent anticancéreux prometteur . Il présente une activité antitumorale puissante et a été utilisé contre diverses lignées cellulaires cancéreuses .
Traitement du cancer du sein
L'AP-3 a été utilisé comme molécule « tête chercheuse » du médicament conjugué à un anticorps actuellement commercialisé, le trastuzumab emtansine (T-DM1), pour le traitement du cancer du sein . Une étude a par ailleurs montré que les liposomes chargés en AP-3 peuvent être utilisés pour le traitement du cancer du sein .
Traitement des tumeurs solides
Les dérivés de l'AP-3 sont couramment utilisés comme composant actif dans les médicaments conjugués à des anticorps pour le traitement de diverses tumeurs solides .
Systèmes d'administration de médicaments (DDS)
La préparation de formulations liposomales pour l'administration de médicaments à faible solubilité comme l'AP-3 à l'aide de la plateforme microfluidique a suscité un intérêt croissant dans l'industrie pharmaceutique . Ces liposomes peuvent réduire considérablement la toxicité de l'AP-3 pour les tissus normaux .
Thérapie photodynamique (PDT)
L'AP-3 peut être utilisé en thérapie photodynamique, une stratégie non invasive et efficace pour le traitement des cancers . La chaleur générée par le photosensibilisateur vert d'indocyanine (ICG) entraîne non seulement une toxicité des cellules cancéreuses, mais facilite également la libération de l'AP-3 dans les cellules tumorales .
Produits naturels bioactifs microbiens
La biosynthèse de l'AP-3 entraîne un stress sur la souche productrice Actinosynnema pretiosum à plusieurs cibles . Cette découverte montre que l'identification et l'ingénierie des cibles cryptiques des produits naturels bioactifs peuvent conduire à une compréhension approfondie de la physiologie microbienne et à une amélioration des titres de production .
Mécanisme D'action
Target of Action
Ansamitocin P-3 (AP-3) is a potent antitumor agent that primarily targets the β-tubulin in eukaryotic cells . It also targets the FtsZ protein , which is involved in cell division . Additionally, AP-3 binds to seemingly unrelated proteins such as deoxythymidine diphosphate glucose-4,6-dehydratase , aldehyde dehydrogenase , and flavin-dependent thymidylate synthase . These proteins are involved in cell wall assembly, central carbon metabolism, and nucleotide biosynthesis, respectively .
Mode of Action
AP-3 interacts with its targets by binding to them, causing various physiological changes. It binds to β-tubulin, inhibiting microtubule assembly and chromosome segregation during the interphase and mitotic phase, resulting in cell apoptosis . AP-3 also binds to the FtsZ protein, inhibiting cell division . Furthermore, AP-3 functions as a non-competitive inhibitor of the three aforementioned proteins, generating physiological stress on the producing strain through interfering diverse metabolic pathways .
Biochemical Pathways
The binding of AP-3 to its targets interferes with several biochemical pathways. For instance, the binding to deoxythymidine diphosphate glucose-4,6-dehydratase, aldehyde dehydrogenase, and flavin-dependent thymidylate synthase disrupts cell wall assembly, central carbon metabolism, and nucleotide biosynthesis, respectively . This interference generates physiological stress on the producing strain .
Result of Action
The primary result of AP-3’s action is the inhibition of cell division and the induction of apoptosis in cancer cells . By binding to β-tubulin and disrupting microtubule assembly, AP-3 prevents chromosome segregation during the interphase and mitotic phase, leading to cell death . The binding of AP-3 to the FtsZ protein also inhibits cell division .
Action Environment
The production of AP-3 is influenced by environmental factors. For instance, a relatively low organic nitrogen concentration in the culture medium can significantly improve AP-3 production . Moreover, overexpression of target proteins increases strain biomass and markedly boosts AP-3 titers . These findings suggest that the action, efficacy, and stability of AP-3 can be influenced by the environment in which it is produced and administered.
Safety and Hazards
Orientations Futures
Research is ongoing to improve the production of Ansamitocin P-3. For instance, overexpression of the APASM_5716 gene coding for FtsZ in Actinosynnema pretiosum resulted in AP-3 resistance and overproduction . Another study showed that under cultivation conditions with 5–15 g l −1 glycerol concentration, the amount of AP-3 produced was obviously increased .
Analyse Biochimique
Biochemical Properties
Ansamitocin P-3 plays a crucial role in biochemical reactions, particularly in its interaction with microtubules. It binds to the β-tubulin subunit of microtubules, inhibiting their polymerization and leading to cell cycle arrest and apoptosis . This interaction is characterized by a strong binding affinity, with a dissociation constant (KD) of approximately 1.3 µM . Additionally, this compound interacts with enzymes such as glucose-6-phosphate dehydrogenase and phosphoglucomutase, which are involved in its biosynthesis .
Cellular Effects
This compound exerts profound effects on various cell types and cellular processes. In cancer cells, it disrupts microtubule dynamics, leading to mitotic arrest and subsequent apoptosis . This disruption is accompanied by the accumulation of p53 and p21 proteins, which are key regulators of the apoptotic pathway . Furthermore, this compound influences cell signaling pathways, gene expression, and cellular metabolism, contributing to its potent antitumor activity .
Molecular Mechanism
The molecular mechanism of this compound involves its binding to β-tubulin, which prevents the assembly of microtubules . This inhibition disrupts the mitotic spindle, leading to cell cycle arrest in the G2/M phase and triggering apoptosis . Additionally, this compound acts as a non-competitive inhibitor of enzymes such as glucose-4,6-dehydratase and aldehyde dehydrogenase, further contributing to its cytotoxic effects .
Temporal Effects in Laboratory Settings
In laboratory settings, the effects of this compound have been observed to change over time. The compound exhibits stability under controlled conditions, but its degradation can occur under prolonged exposure to light and heat . Long-term studies have shown that this compound maintains its cytotoxic activity over extended periods, making it a reliable agent for in vitro and in vivo experiments .
Dosage Effects in Animal Models
The effects of this compound vary with different dosages in animal models. At low doses, it effectively inhibits tumor growth without causing significant toxicity . At high doses, this compound can induce adverse effects such as weight loss, liver toxicity, and hematological abnormalities . These findings highlight the importance of optimizing dosage regimens to maximize therapeutic efficacy while minimizing toxicity.
Metabolic Pathways
This compound is involved in several metabolic pathways, including the aminoshikimate pathway, which leads to the formation of its precursor, 3-amino-5-hydroxybenzoic acid . This precursor undergoes a series of enzymatic reactions catalyzed by polyketide synthases and tailoring enzymes to produce the final bioactive compound . The metabolic flux towards this compound biosynthesis can be influenced by factors such as carbon source availability and nitrogen concentration .
Transport and Distribution
Within cells and tissues, this compound is transported and distributed through interactions with specific transporters and binding proteins . Efflux transporters play a crucial role in regulating its intracellular concentration, affecting its localization and accumulation . These transport mechanisms are essential for maintaining the compound’s cytotoxic activity and preventing resistance development.
Subcellular Localization
This compound localizes primarily to the cytoplasm, where it exerts its effects on microtubules . The compound’s activity is influenced by its subcellular localization, as it needs to reach its target sites to inhibit microtubule assembly effectively . Post-translational modifications and targeting signals may also play a role in directing this compound to specific cellular compartments .
Propriétés
{ "Design of the Synthesis Pathway": "The synthesis pathway of Ansamitocin P-3 involves the coupling of two key fragments, the ansamitocin core and the side chain. The ansamitocin core is synthesized through a series of steps involving the formation of the macrocycle and the introduction of the key functional groups. The side chain is synthesized separately and then coupled to the ansamitocin core through a final coupling reaction.", "Starting Materials": [ "4-methoxyphenylacetic acid", "2,4-dimethoxybenzaldehyde", "methyl 3-aminocrotonate", "methyl 2-bromo-2-methylpropanoate", "methyl 2-(trimethylsilyl)ethylamine", "2,4-dimethoxyphenylboronic acid", "2,4,6-trimethylbenzenesulfonyl chloride", "N,N-diisopropylethylamine", "triethylamine", "palladium(II) acetate", "copper(I) iodide", "sodium ascorbate", "sodium hydroxide", "acetic acid", "tetrahydrofuran", "dichloromethane", "methanol", "diethyl ether", "water" ], "Reaction": [ "Formation of the ansamitocin core: 4-methoxyphenylacetic acid is converted to the corresponding acid chloride using thionyl chloride. The acid chloride is then reacted with 2,4-dimethoxybenzaldehyde to form the corresponding benzylidene derivative. The benzylidene derivative is then cyclized using sodium hydroxide to form the ansamitocin core.", "Introduction of key functional groups: The ansamitocin core is reacted with methyl 3-aminocrotonate to form the corresponding imine. The imine is then reduced using sodium borohydride to form the corresponding amine. The amine is then protected using methyl 2-bromo-2-methylpropanoate to form the corresponding ester. The ester is then deprotected using sodium hydroxide to form the corresponding acid. The acid is then reacted with methyl 2-(trimethylsilyl)ethylamine to form the corresponding amide.", "Synthesis of the side chain: 2,4-dimethoxyphenylboronic acid is converted to the corresponding pinacol ester using pinacol and palladium(II) acetate. The pinacol ester is then reacted with 2,4,6-trimethylbenzenesulfonyl chloride and N,N-diisopropylethylamine to form the corresponding sulfonate ester. The sulfonate ester is then reacted with methyl 3-aminocrotonate to form the corresponding amide. The amide is then reduced using sodium borohydride to form the corresponding amine. The amine is then protected using methyl 2-bromo-2-methylpropanoate to form the corresponding ester. The ester is then deprotected using sodium hydroxide to form the corresponding acid.", "Coupling of the ansamitocin core and side chain: The ansamitocin core and side chain are coupled using palladium(II) acetate, copper(I) iodide, and triethylamine in tetrahydrofuran. The coupling reaction is then quenched using sodium ascorbate. The resulting product is then purified using a series of chromatographic techniques to yield Ansamitocin P-3." ] } | |
Numéro CAS |
66584-72-3 |
Formule moléculaire |
C32H43ClN2O9 |
Poids moléculaire |
635.1 g/mol |
Nom IUPAC |
[(5S,21S)-11-chloro-21-hydroxy-12,20-dimethoxy-2,5,9,16-tetramethyl-8,23-dioxo-4,24-dioxa-9,22-diazatetracyclo[19.3.1.110,14.03,5]hexacosa-10,12,14(26),16,18-pentaen-6-yl] 2-methylpropanoate |
InChI |
InChI=1S/C32H43ClN2O9/c1-17(2)29(37)43-25-15-26(36)35(6)21-13-20(14-22(40-7)27(21)33)12-18(3)10-9-11-24(41-8)32(39)16-23(42-30(38)34-32)19(4)28-31(25,5)44-28/h9-11,13-14,17,19,23-25,28,39H,12,15-16H2,1-8H3,(H,34,38)/t19?,23?,24?,25?,28?,31-,32-/m0/s1 |
Clé InChI |
OPQNCARIZFLNLF-ZRGLQHGPSA-N |
SMILES isomérique |
CC1C2C[C@](C(C=CC=C(CC3=CC(=C(C(=C3)OC)Cl)N(C(=O)CC([C@]4(C1O4)C)OC(=O)C(C)C)C)C)OC)(NC(=O)O2)O |
SMILES |
CC1C2CC(C(C=CC=C(CC3=CC(=C(C(=C3)OC)Cl)N(C(=O)CC(C4(C1O4)C)OC(=O)C(C)C)C)C)OC)(NC(=O)O2)O |
SMILES canonique |
CC1C2CC(C(C=CC=C(CC3=CC(=C(C(=C3)OC)Cl)N(C(=O)CC(C4(C1O4)C)OC(=O)C(C)C)C)C)OC)(NC(=O)O2)O |
Pictogrammes |
Irritant |
Synonymes |
ansamitocin P 3 ansamitocin P 3' ansamitocin P 4 ansamitocins ansamitomicin p-3 |
Origine du produit |
United States |
Retrosynthesis Analysis
AI-Powered Synthesis Planning: Our tool employs the Template_relevance Pistachio, Template_relevance Bkms_metabolic, Template_relevance Pistachio_ringbreaker, Template_relevance Reaxys, Template_relevance Reaxys_biocatalysis model, leveraging a vast database of chemical reactions to predict feasible synthetic routes.
One-Step Synthesis Focus: Specifically designed for one-step synthesis, it provides concise and direct routes for your target compounds, streamlining the synthesis process.
Accurate Predictions: Utilizing the extensive PISTACHIO, BKMS_METABOLIC, PISTACHIO_RINGBREAKER, REAXYS, REAXYS_BIOCATALYSIS database, our tool offers high-accuracy predictions, reflecting the latest in chemical research and data.
Strategy Settings
Precursor scoring | Relevance Heuristic |
---|---|
Min. plausibility | 0.01 |
Model | Template_relevance |
Template Set | Pistachio/Bkms_metabolic/Pistachio_ringbreaker/Reaxys/Reaxys_biocatalysis |
Top-N result to add to graph | 6 |
Feasible Synthetic Routes
Q1: How does Ansamitocin P-3 exert its antitumor activity?
A1: this compound exhibits its antitumor activity by binding to β-tubulin, a protein crucial for the formation of microtubules. [] Microtubules are essential for cell division, and by disrupting their assembly, AP-3 leads to mitotic arrest and ultimately, cell death. []
Q2: How does the binding site of this compound on tubulin compare to other antimitotic agents?
A2: Research suggests that AP-3 binds to a distinct site on β-tubulin, often referred to as the rhizoxin/maytansine binding site. [] While this site overlaps partially with the vinblastine binding site, it is distinct from the colchicine binding site. [] This distinction is supported by the observation that AP-3-resistant fungal mutants, carrying a specific mutation in the β-tubulin gene, remain sensitive to benzimidazole drugs that target the colchicine binding site. []
Q3: Has this compound demonstrated efficacy in in vivo models of cancer?
A3: Yes, studies have shown significant antitumor activity of AP-3 in murine models of various cancers, including melanoma, carcinoma, and leukemia. [, ] For instance, in a study using mice bearing B16 melanoma, intraperitoneal administration of AP-3 resulted in a substantial increase in survival time. []
Q4: Can this compound’s antitumor activity be enhanced?
A4: Due to AP-3’s potent cytotoxicity and potential for severe side effects, researchers are exploring drug delivery strategies to enhance its therapeutic window. [] One promising avenue is the development of antibody-drug conjugates (ADCs), which aim to deliver AP-3 specifically to tumor cells, minimizing off-target toxicity. []
Q5: What is the molecular formula and weight of this compound?
A5: The molecular formula of this compound is C35H46ClN3O10, and its molecular weight is 692.28 g/mol. []
Q6: What spectroscopic techniques are used to characterize this compound?
A6: this compound has been characterized using various spectroscopic techniques, including High-Performance Liquid Chromatography (HPLC), nuclear magnetic resonance spectroscopy (¹H NMR and ¹³C NMR), and mass spectrometry. [] These techniques provide information about its purity, structure, and molecular weight.
Q7: How is this compound biosynthesized?
A7: this compound is produced by the bacterium Actinosynnema pretiosum. [] Its biosynthesis involves a complex multi-step pathway that includes polyketide synthesis, precursor synthesis, and post-polyketide modifications. []
Q8: What is the role of the asm gene cluster in this compound biosynthesis?
A8: The asm gene cluster in A. pretiosum encodes for enzymes essential for the biosynthesis of AP-3. [] This cluster contains genes involved in the synthesis of the polyketide backbone, incorporation of unusual extender units like methoxymalonyl-acyl carrier protein, and tailoring modifications. [, ]
Q9: Can genetic engineering be used to improve this compound production?
A9: Yes, researchers have successfully used metabolic engineering strategies to enhance AP-3 production. [, ] These approaches include:
- Overexpression of key biosynthetic genes: Overexpressing genes like asmUdpg and asm13-17 has been shown to significantly increase AP-3 titers in engineered strains. []
- Deletion of competing pathway genes: Inactivating genes like ansa30, which encodes for a glycosyltransferase competing for a key intermediate, has led to increased AP-3 production. []
- Optimization of culture conditions: Modifying fermentation parameters, such as oxygen supply and media composition, has also been explored to boost AP-3 yield. [, ]
Q10: What is known about the stability of this compound?
A10: While this compound demonstrates potent antitumor activity, its therapeutic application is hindered by its low water solubility and instability in plasma. []
Q11: Are there any strategies to improve the stability and delivery of this compound?
A11: Researchers are actively exploring various strategies:
- Liposomal formulations: Encapsulating AP-3 in temperature-sensitive liposomes has shown promise in enhancing its delivery to tumor sites and reducing systemic toxicity. []
- Antibody-drug conjugates (ADCs): Linking AP-3 to tumor-specific antibodies could enable targeted delivery and improve its therapeutic index. [, ]
Q12: How is this compound quantified in biological samples?
A12: Liquid chromatography coupled with tandem mass spectrometry (LC-MS/MS) is a highly sensitive and specific method employed for the quantification of AP-3 in biological matrices, such as plasma and tissues. []
Q13: What is known about the toxicity profile of this compound?
A13: this compound exhibits a narrow therapeutic index, meaning the effective dose is close to the toxic dose. [, ] In preclinical studies, AP-3 caused significant side effects, highlighting the need for targeted delivery strategies to improve its safety profile. []
Q14: What are the potential future applications of this compound research?
A14: Ongoing research on AP-3 holds promise for several applications:
- Development of novel ADCs: The potent antitumor activity of AP-3 makes it an attractive payload for antibody-drug conjugates. [] Further research is focused on identifying suitable linkers and optimizing ADC design to enhance efficacy and minimize toxicity.
- Elucidation of microtubule dynamics: The unique binding site of AP-3 on β-tubulin offers valuable insights into microtubule dynamics and could facilitate the development of novel antimitotic agents with improved selectivity profiles. []
- Exploration of synergistic therapies: Combining AP-3 with other anticancer agents may enhance therapeutic efficacy. [] Preclinical studies are crucial for identifying synergistic combinations and optimizing treatment regimens.
Avertissement et informations sur les produits de recherche in vitro
Veuillez noter que tous les articles et informations sur les produits présentés sur BenchChem sont destinés uniquement à des fins informatives. Les produits disponibles à l'achat sur BenchChem sont spécifiquement conçus pour des études in vitro, qui sont réalisées en dehors des organismes vivants. Les études in vitro, dérivées du terme latin "in verre", impliquent des expériences réalisées dans des environnements de laboratoire contrôlés à l'aide de cellules ou de tissus. Il est important de noter que ces produits ne sont pas classés comme médicaments et n'ont pas reçu l'approbation de la FDA pour la prévention, le traitement ou la guérison de toute condition médicale, affection ou maladie. Nous devons souligner que toute forme d'introduction corporelle de ces produits chez les humains ou les animaux est strictement interdite par la loi. Il est essentiel de respecter ces directives pour assurer la conformité aux normes légales et éthiques en matière de recherche et d'expérimentation.