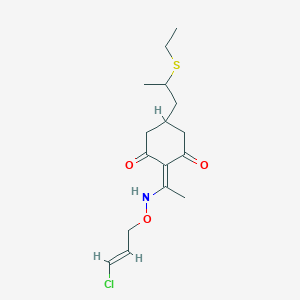
C-Demethyl Clethodim
- Cliquez sur DEMANDE RAPIDE pour recevoir un devis de notre équipe d'experts.
- Avec des produits de qualité à un prix COMPÉTITIF, vous pouvez vous concentrer davantage sur votre recherche.
Vue d'ensemble
Description
C-Demethyl Clethodim is a yellow to brown solid and a metabolite of Clethodim, a selective herbicide. It is primarily used in agricultural settings to control a variety of broadleaf weeds and some grass crops . The compound is known for its high selectivity and effectiveness in weed control.
Méthodes De Préparation
Synthetic Routes and Reaction Conditions: The synthesis of C-Demethyl Clethodim involves several steps, starting from Clethodim. The process typically includes the demethylation of Clethodim under specific reaction conditions. The exact synthetic route and reaction conditions are proprietary and may vary depending on the manufacturer .
Industrial Production Methods: Industrial production of this compound involves large-scale chemical synthesis using high-performance liquid chromatography (HPLC) for purification . The process is designed to ensure high yield and purity of the final product.
Analyse Des Réactions Chimiques
Types of Reactions: C-Demethyl Clethodim undergoes various chemical reactions, including oxidation, reduction, and substitution. These reactions are essential for its function as a herbicide.
Common Reagents and Conditions: Common reagents used in the reactions involving this compound include oxidizing agents, reducing agents, and various solvents. The conditions for these reactions are typically controlled to ensure the desired outcome .
Major Products Formed: The major products formed from the reactions of this compound include various metabolites that contribute to its herbicidal activity. These metabolites are crucial for its effectiveness in weed control .
Applications De Recherche Scientifique
Herbicidal Applications
C-Demethyl Clethodim is primarily applied in:
- Post-emergent Weed Control : It is effective against annual and perennial grass weeds in various crops, including soybeans, cotton, and sugar beets. The compound selectively targets grasses while being safe for dicot crops, making it a valuable tool in integrated weed management systems .
- Mechanism of Action : The herbicidal activity of this compound involves the chelation of metal ions associated with plant enzymes, disrupting essential biochemical processes necessary for plant growth . This selectivity is critical for maintaining crop health while controlling unwanted vegetation.
Metabolism and Residue Studies
Understanding the metabolism of this compound is essential for assessing its environmental impact and safety:
- Metabolic Pathways : Research indicates that this compound undergoes extensive metabolism in treated crops. Major metabolites include clethodim sulfoxide and clethodim sulfone, which are often not detected in mature crops . This degradation pathway is significant for evaluating maximum residue levels (MRLs) in food products.
- Residue Levels : Studies have shown that when used according to good agricultural practices (GAPs), residues of this compound are expected to remain below MRLs, ensuring consumer safety . The European Food Safety Authority (EFSA) emphasizes the need for ongoing research to fully understand the implications of different isomer ratios present in this compound applications .
Case Studies and Research Findings
Several case studies highlight the practical applications and effectiveness of this compound:
- Field Efficacy Trials : In trials conducted across various crops, this compound demonstrated effective control of resistant grass species. For example, a study showed that despite resistance to other ACCase-inhibiting herbicides, populations of Lolium rigidum remained susceptible to this compound at recommended application rates .
- Environmental Safety Assessments : Risk assessments conducted by regulatory bodies such as the EPA indicate that this compound poses low toxicity risks to humans and non-target organisms when applied correctly. The primary effects observed were on liver health in test animals, but these were determined to be within acceptable limits for agricultural use .
Comparative Effectiveness
To illustrate the effectiveness of this compound compared to other herbicides, the following table summarizes key findings from various studies:
Herbicide | Target Weeds | Application Rate (lbs/acre) | Efficacy (%) | Notes |
---|---|---|---|---|
This compound | Annual & Perennial Grasses | 0.006 - 0.19 | 85 - 95 | Effective on resistant populations |
Haloxyfop | Grasses | 0.5 - 1.0 | 70 - 80 | Resistance issues reported |
Sethoxydim | Grasses | 0.5 - 1.0 | 75 - 85 | Less effective on certain resistant types |
Mécanisme D'action
C-Demethyl Clethodim exerts its effects by inhibiting acetyl coenzyme A carboxylase, an enzyme involved in fatty acid biosynthesis. This inhibition disrupts lipid synthesis, leading to the death of targeted weeds . The compound interacts with specific molecular targets and pathways, making it highly effective as a herbicide.
Comparaison Avec Des Composés Similaires
Similar Compounds: Similar compounds to C-Demethyl Clethodim include other cyclohexanedione oximes such as Clethodim, Sethoxydim, and Tralkoxydim .
Uniqueness: this compound is unique due to its high selectivity and effectiveness in controlling a wide range of weeds. Its specific mechanism of action and the ability to target specific molecular pathways make it a valuable tool in agricultural weed management .
Activité Biologique
C-Demethyl Clethodim, a derivative of the herbicide Clethodim, exhibits significant biological activity primarily through its role as an inhibitor of the enzyme acetyl-CoA carboxylase (ACCase). This compound is utilized in agricultural practices to control grass weeds in various crops. Understanding its biological activity is crucial for assessing its efficacy and safety in both agricultural and ecological contexts.
Target Enzyme:
this compound specifically targets acetyl-CoA carboxylase , an enzyme integral to fatty acid biosynthesis in plants. By inhibiting this enzyme, the compound disrupts lipid synthesis, ultimately leading to phytotoxic effects such as stunted growth and reduced germination rates in susceptible plant species.
Mode of Action:
The herbicidal activity is characterized by the selective inhibition of ACCase, which is vital for the formation of cell membranes. This inhibition results in the cessation of new cell growth and promotes gradual plant death .
Pharmacokinetics
This compound undergoes a series of metabolic transformations after application. The pharmacokinetic profile includes:
- Absorption: Rapid absorption into plant tissues.
- Metabolism: Conversion into various metabolites, including clethodim sulfoxide and hydroxylated forms.
- Excretion: The compound and its metabolites are eventually excreted from the plant system.
This compound interacts with multiple biomolecules, affecting cellular processes. It has been shown to induce oxidative stress in cells, leading to cytotoxic effects. In laboratory studies, it has demonstrated significant inhibition of cellular proliferation in liver cell lines at varying concentrations:
- IC50 Values:
- B129 mouse liver cells: 220 µg/ml
- THLE-2 human liver cells: 617 µg/ml
These findings indicate a higher sensitivity of mouse liver cells compared to human liver cells when exposed to this compound .
Cellular Effects
The compound exhibits notable phytotoxicity , which manifests as:
- Inhibition of seed germination.
- Reduction in root growth.
- Dose-dependent decrease in cell viability in cultured liver cells.
In laboratory settings, concentrations above 800 µg/ml significantly compromised cell shape and viability .
Case Studies and Research Findings
-
Study on Cytotoxic Effects:
A study evaluated the cytotoxic effects of clethodim on different liver cell lines. The results indicated that high doses led to substantial reductions in cell number and viability, particularly in mouse liver cells compared to human cells . -
Resistance Mechanisms:
Research has identified mutations in ACCase that confer resistance to clethodim among certain weed populations. These mutations highlight the evolutionary adaptations that can occur in response to herbicide application, emphasizing the need for integrated weed management strategies . -
Toxicological Evaluations:
Toxicological assessments have shown that repeated exposure to clethodim primarily affects the liver across various animal models. Observed effects include increased liver weights and hepatic hypertrophy, suggesting potential implications for long-term exposure risks .
Propriétés
IUPAC Name |
2-[(E)-N-[(E)-3-chloroprop-2-enoxy]-C-methylcarbonimidoyl]-5-(2-ethylsulfanylpropyl)-3-hydroxycyclohex-2-en-1-one |
Source
|
---|---|---|
Source | PubChem | |
URL | https://pubchem.ncbi.nlm.nih.gov | |
Description | Data deposited in or computed by PubChem | |
InChI |
InChI=1S/C16H24ClNO3S/c1-4-22-11(2)8-13-9-14(19)16(15(20)10-13)12(3)18-21-7-5-6-17/h5-6,11,13,19H,4,7-10H2,1-3H3/b6-5+,18-12+ |
Source
|
Source | PubChem | |
URL | https://pubchem.ncbi.nlm.nih.gov | |
Description | Data deposited in or computed by PubChem | |
InChI Key |
YFVJEPZJOFFBPS-YADWXFRQSA-N |
Source
|
Source | PubChem | |
URL | https://pubchem.ncbi.nlm.nih.gov | |
Description | Data deposited in or computed by PubChem | |
Canonical SMILES |
CCSC(C)CC1CC(=C(C(=O)C1)C(=NOCC=CCl)C)O |
Source
|
Source | PubChem | |
URL | https://pubchem.ncbi.nlm.nih.gov | |
Description | Data deposited in or computed by PubChem | |
Isomeric SMILES |
CCSC(C)CC1CC(=C(C(=O)C1)/C(=N/OC/C=C/Cl)/C)O |
Source
|
Source | PubChem | |
URL | https://pubchem.ncbi.nlm.nih.gov | |
Description | Data deposited in or computed by PubChem | |
Molecular Formula |
C16H24ClNO3S |
Source
|
Source | PubChem | |
URL | https://pubchem.ncbi.nlm.nih.gov | |
Description | Data deposited in or computed by PubChem | |
Molecular Weight |
345.9 g/mol |
Source
|
Source | PubChem | |
URL | https://pubchem.ncbi.nlm.nih.gov | |
Description | Data deposited in or computed by PubChem | |
Avertissement et informations sur les produits de recherche in vitro
Veuillez noter que tous les articles et informations sur les produits présentés sur BenchChem sont destinés uniquement à des fins informatives. Les produits disponibles à l'achat sur BenchChem sont spécifiquement conçus pour des études in vitro, qui sont réalisées en dehors des organismes vivants. Les études in vitro, dérivées du terme latin "in verre", impliquent des expériences réalisées dans des environnements de laboratoire contrôlés à l'aide de cellules ou de tissus. Il est important de noter que ces produits ne sont pas classés comme médicaments et n'ont pas reçu l'approbation de la FDA pour la prévention, le traitement ou la guérison de toute condition médicale, affection ou maladie. Nous devons souligner que toute forme d'introduction corporelle de ces produits chez les humains ou les animaux est strictement interdite par la loi. Il est essentiel de respecter ces directives pour assurer la conformité aux normes légales et éthiques en matière de recherche et d'expérimentation.