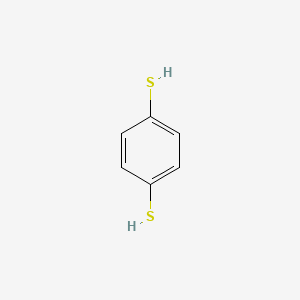
1,4-Benzenedithiol
Vue d'ensemble
Description
Synthesis Analysis
The synthesis of 1,4-Benzenedithiol has been explored through various methods, including the reaction of methyl mercaptan sodium with o-dichlorobenzene in DMF followed by acidic hydrolysis, optimizing the route for industrial production. The yield of 1,2-benzenedithiol reached up to 93% under optimal conditions, demonstrating the efficiency of this synthesis method (Guo-tong, 2010).
Molecular Structure Analysis
The crystal structure of this compound has been determined using the Rietveld method and the Molecular Mechanics Program (MMP2), revealing a monoclinic P 21 /c structure. This structure facilitates the understanding of the solid-state addition polymerization mechanism of BDT to 1,4-diethynylbenzene (DEB), with DEB columns situated between BDT columns, allowing for addition polymerization under UV irradiation (Ohashi et al., 1997).
Chemical Reactions and Properties
BDT's reactivity has been studied through its interactions with metal fragments, demonstrating its role as an "innocent" ligand in complexes containing [M(S2)2] fragments. This reactivity indicates BDT's potential in forming stable, high-conductance molecular junctions suitable for molecular device applications (Sellmann et al., 2004).
Physical Properties Analysis
The conductance of single BDT molecules has been investigated, showing clear conductance steps in both low and high-conductance regimes. This indicates distinct conductance states attributable to different Au–S bonding configurations, with the high-conductance state being stable up to 1.6V, underscoring its potential for molecular device applications (Tsutsui et al., 2006).
Chemical Properties Analysis
Studies on the adsorption behavior of BDT on colloidal gold and silver surfaces through surface-enhanced Raman scattering (SERS) have provided insights into its chemisorption as mono- and dithiolate. These findings contribute to understanding the surface chemistry of BDT, essential for its applications in molecular electronics and sensing (Joo et al., 2001).
Applications De Recherche Scientifique
Molecular Electronics and Charge Transport
- BDT is widely studied in molecular electronics, particularly in molecular junctions where it's used to explore the interplay between electrical and mechanical properties of molecules. For instance, stretching a BDT molecular junction can increase conductance significantly, shedding light on the strain-induced shift of molecular orbitals and resonant enhancement of conductance (Bruot, Hihath, & Tao, 2011).
- The conductance properties of BDT have been further studied by examining the effect of anchoring group position on the formation and conductance of single molecule junctions, providing insights into conductive molecular orbitals and electron pathways (Kiguchi et al., 2010).
Surface-Enhanced Raman Spectroscopy (SERS)
- BDT is significant in the study of SERS due to its benzene ring structure and sulfhydryl group, which aid in adsorption on metal nanostructures. This has implications for the quantitative analysis of BDT as a Raman probe molecule (Lu, 2022).
- Density functional theory (DFT) studies have been conducted on BDT and its complexes to understand surface-enhanced Raman chemical enhancement mechanisms, providing insights into the interaction between Raman intensity, molecular properties, and excited states (Shao et al., 2014).
Organic Materials in Energy Storage
- BDT derivatives like Lithium benzenedithiolate have been explored as potential high-capacity organic cathode materials for rechargeable lithium batteries, with studies focusing on their redox chemistry and electrochemical behavior (Li et al., 2019).
Catalysis and Chemical Reactions
- BDT has been found to accelerate Fenton reactions for dye degradation, offering insights into its role as a co-catalyst and its effects on the Fe3+/Fe2+ cycle (Ma Jia, 2012).
- The use of BDT in the synthesis and characterization of metal-organic polymers has been explored, revealing insights into their structure and potential applications in conductivity studies (Turner et al., 2012).
Biomedical Applications
- BDT-bridged core-satellite assemblies have been employed as SERS mechanical probes for monitoring endocytic forces in live cells, providing a method to visualize and discriminate between different cell types (Feng et al., 2022).
Other Applications
- The sonolytic hydrolysis of peptides with the addition of 1,4-BDT has been investigated, demonstrating improvements in the rate of hydrolysis reaction and providing insights into the mechanistic reaction of peptide hydrolysis (Sakakura & Takayama, 2012).
Mécanisme D'action
Target of Action
1,4-Benzenedithiol (BDT) is an organic sulfur compound . It primarily targets gold (Au) electrodes and is used in single molecule junctions in electronic devices . The strong Au-S bond formed between the BDT molecule and the Au electrode facilitates high electron transmission through the molecule-electrode interface .
Mode of Action
BDT interacts with its targets (Au electrodes) by forming a bridge between two electrodes . This interaction is facilitated by the strong Au-S bond, which results in high electron transmission through the molecule-electrode interface . The electron transmission characteristics of Au/BDT/Au junctions have been studied extensively, revealing clear conductance steps in both low and high-conductance regimes .
Biochemical Pathways
It’s known that bdt can greatly accelerate the fenton reaction of dyes such as malachite green and rhodamine b . This suggests that BDT may influence redox reactions and related biochemical pathways.
Pharmacokinetics
It’s known that bdt is a white to yellow crystalline solid with a strong sulfur odor . It has low volatility at room temperature and is slightly soluble in water but has higher solubility in
Safety and Hazards
1,4-Benzenedithiol is considered a highly toxic chemical and can have serious health effects if ingested, inhaled, or absorbed through the skin. It is known to irritate the skin, eyes, and respiratory system. Long-term exposure can lead to organ damage and even cancer . It is advised to avoid dust formation, avoid breathing mist, gas or vapors, avoid contacting with skin and eye, and use personal protective equipment .
Orientations Futures
The high-conductance state of single BDT molecules is stable up to 1.6 V and is prospective for molecular device applications . These nanoscale molecular electronic networks linked via metallic clusters provide an avenue for engineering electronics at the molecular level by using superstructures of different organic molecules and topologies .
Analyse Biochimique
Biochemical Properties
1,4-Benzenedithiol plays a significant role in biochemical reactions, particularly in the formation of self-assembled monolayers (SAMs) on various surfaces . It interacts with enzymes, proteins, and other biomolecules through its thiol groups, which can form strong covalent bonds with metal ions and other thiol-reactive species. This interaction is crucial in the development of nanomaterials and electronic devices . Additionally, this compound can act as a cross-linker in the synthesis of polymers and other complex molecules .
Cellular Effects
This compound has been shown to influence various cellular processes. It can accelerate the Fenton reaction, which is involved in the degradation of organic dyes such as malachite green and Rhodamine B . This acceleration is due to the compound’s ability to facilitate the redox cycling of iron ions, thereby enhancing the production of reactive oxygen species (ROS). These ROS can affect cell signaling pathways, gene expression, and cellular metabolism . The compound’s impact on cellular function can vary depending on the concentration and exposure duration.
Molecular Mechanism
At the molecular level, this compound exerts its effects through binding interactions with biomolecules. It can form covalent bonds with metal ions, such as gold, which is utilized in the fabrication of nanomaterials and electronic devices . The compound’s thiol groups can also interact with proteins and enzymes, leading to enzyme inhibition or activation. For example, this compound can inhibit the activity of certain enzymes by binding to their active sites, thereby preventing substrate access . Additionally, the compound can modulate gene expression by affecting transcription factors and other regulatory proteins.
Temporal Effects in Laboratory Settings
In laboratory settings, the effects of this compound can change over time. The compound is relatively stable under standard conditions, but it can degrade when exposed to light, heat, or oxidative environments . Long-term exposure to this compound can lead to alterations in cellular function, including changes in gene expression and metabolic activity. These temporal effects are important to consider when designing experiments and interpreting results.
Dosage Effects in Animal Models
The effects of this compound can vary with different dosages in animal models. At low doses, the compound may have minimal impact on cellular function, while higher doses can lead to toxic or adverse effects . For example, high doses of this compound can cause oxidative stress, leading to cell damage and apoptosis. It is important to determine the appropriate dosage range to avoid potential toxicity and ensure the desired biochemical effects.
Metabolic Pathways
This compound is involved in various metabolic pathways, particularly those related to redox reactions and thiol metabolism . The compound can interact with enzymes such as glutathione reductase and thioredoxin reductase, which play key roles in maintaining cellular redox balance. These interactions can affect metabolic flux and alter the levels of metabolites involved in redox homeostasis .
Transport and Distribution
Within cells and tissues, this compound is transported and distributed through interactions with transporters and binding proteins . The compound’s thiol groups can bind to metal ions and other thiol-reactive species, facilitating its transport across cell membranes and its accumulation in specific cellular compartments. This transport and distribution are crucial for the compound’s biochemical and cellular effects.
Subcellular Localization
This compound’s subcellular localization is influenced by its chemical properties and interactions with biomolecules . The compound can localize to specific cellular compartments, such as the cytoplasm, nucleus, or mitochondria, depending on the presence of targeting signals or post-translational modifications. This localization can affect the compound’s activity and function, as well as its interactions with other biomolecules.
Propriétés
IUPAC Name |
benzene-1,4-dithiol | |
---|---|---|
Source | PubChem | |
URL | https://pubchem.ncbi.nlm.nih.gov | |
Description | Data deposited in or computed by PubChem | |
InChI |
InChI=1S/C6H6S2/c7-5-1-2-6(8)4-3-5/h1-4,7-8H | |
Source | PubChem | |
URL | https://pubchem.ncbi.nlm.nih.gov | |
Description | Data deposited in or computed by PubChem | |
InChI Key |
WYLQRHZSKIDFEP-UHFFFAOYSA-N | |
Source | PubChem | |
URL | https://pubchem.ncbi.nlm.nih.gov | |
Description | Data deposited in or computed by PubChem | |
Canonical SMILES |
C1=CC(=CC=C1S)S | |
Source | PubChem | |
URL | https://pubchem.ncbi.nlm.nih.gov | |
Description | Data deposited in or computed by PubChem | |
Molecular Formula |
C6H6S2 | |
Source | PubChem | |
URL | https://pubchem.ncbi.nlm.nih.gov | |
Description | Data deposited in or computed by PubChem | |
DSSTOX Substance ID |
DTXSID80405615 | |
Record name | 1,4-Benzenedithiol | |
Source | EPA DSSTox | |
URL | https://comptox.epa.gov/dashboard/DTXSID80405615 | |
Description | DSSTox provides a high quality public chemistry resource for supporting improved predictive toxicology. | |
Molecular Weight |
142.2 g/mol | |
Source | PubChem | |
URL | https://pubchem.ncbi.nlm.nih.gov | |
Description | Data deposited in or computed by PubChem | |
CAS RN |
624-39-5 | |
Record name | 1,4-Benzenedithiol | |
Source | CAS Common Chemistry | |
URL | https://commonchemistry.cas.org/detail?cas_rn=624-39-5 | |
Description | CAS Common Chemistry is an open community resource for accessing chemical information. Nearly 500,000 chemical substances from CAS REGISTRY cover areas of community interest, including common and frequently regulated chemicals, and those relevant to high school and undergraduate chemistry classes. This chemical information, curated by our expert scientists, is provided in alignment with our mission as a division of the American Chemical Society. | |
Explanation | The data from CAS Common Chemistry is provided under a CC-BY-NC 4.0 license, unless otherwise stated. | |
Record name | 1,4-Benzenedithiol | |
Source | EPA DSSTox | |
URL | https://comptox.epa.gov/dashboard/DTXSID80405615 | |
Description | DSSTox provides a high quality public chemistry resource for supporting improved predictive toxicology. | |
Record name | 1,4-Benzenedithiol | |
Source | European Chemicals Agency (ECHA) | |
URL | https://echa.europa.eu/information-on-chemicals | |
Description | The European Chemicals Agency (ECHA) is an agency of the European Union which is the driving force among regulatory authorities in implementing the EU's groundbreaking chemicals legislation for the benefit of human health and the environment as well as for innovation and competitiveness. | |
Explanation | Use of the information, documents and data from the ECHA website is subject to the terms and conditions of this Legal Notice, and subject to other binding limitations provided for under applicable law, the information, documents and data made available on the ECHA website may be reproduced, distributed and/or used, totally or in part, for non-commercial purposes provided that ECHA is acknowledged as the source: "Source: European Chemicals Agency, http://echa.europa.eu/". Such acknowledgement must be included in each copy of the material. ECHA permits and encourages organisations and individuals to create links to the ECHA website under the following cumulative conditions: Links can only be made to webpages that provide a link to the Legal Notice page. | |
Retrosynthesis Analysis
AI-Powered Synthesis Planning: Our tool employs the Template_relevance Pistachio, Template_relevance Bkms_metabolic, Template_relevance Pistachio_ringbreaker, Template_relevance Reaxys, Template_relevance Reaxys_biocatalysis model, leveraging a vast database of chemical reactions to predict feasible synthetic routes.
One-Step Synthesis Focus: Specifically designed for one-step synthesis, it provides concise and direct routes for your target compounds, streamlining the synthesis process.
Accurate Predictions: Utilizing the extensive PISTACHIO, BKMS_METABOLIC, PISTACHIO_RINGBREAKER, REAXYS, REAXYS_BIOCATALYSIS database, our tool offers high-accuracy predictions, reflecting the latest in chemical research and data.
Strategy Settings
Precursor scoring | Relevance Heuristic |
---|---|
Min. plausibility | 0.01 |
Model | Template_relevance |
Template Set | Pistachio/Bkms_metabolic/Pistachio_ringbreaker/Reaxys/Reaxys_biocatalysis |
Top-N result to add to graph | 6 |
Feasible Synthetic Routes
Q & A
Q1: How does 1,4-benzenedithiol interact with gold surfaces?
A1: this compound chemisorbs onto gold surfaces by forming strong gold-sulfur bonds. This interaction can occur through one or both sulfur atoms, leading to the formation of monothiolate or dithiolate species, respectively. [] The orientation of 1,4-BDT on the gold surface depends on factors like surface coverage and solvent. []
Q2: Can this compound form self-assembled structures on gold surfaces?
A2: Yes, research shows that 1,4-BDT can self-assemble into oligomeric chains on Au(111) surfaces. [] Initially, η1-thiolate species form, but at room temperature, dithiolates dominate, with the benzene ring nearly parallel to the surface. [] STM imaging reveals zigzag structures, attributed to sulfur hybridization, and hexagonal structures formed by gold adatom-linked 1,4-BDT trimers. []
Q3: How does the presence of gold nanoparticles affect the Raman scattering of this compound?
A3: The Raman scattering of this compound is significantly enhanced in the presence of gold nanoparticles. [, , ] This enhancement is attributed to a combination of electromagnetic and chemical effects. [, , ] Electromagnetic enhancement arises from the localized surface plasmon resonance of the gold nanoparticles, while chemical enhancement is linked to charge transfer between the molecule and the metal surface. [, , ]
Q4: How does the interaction between this compound and gold affect its vibrational properties?
A4: Upon chemisorption to gold surfaces, the vibrational energy and intensity of 1,4-BDT's Raman spectra show distinct changes compared to its bulk form. [] The molecule-metal charge transfer weakens intramolecular bonds, resulting in a red shift of the breathing and C=C stretching modes. [] This effect is more pronounced with multiple molecule-metal interfaces. [] Additionally, new b2 signals, absent in bulk spectra, arise due to chemical enhancement via photoinduced charge transfer. []
Q5: Can this compound act as a bridge in single-molecule junctions?
A5: Yes, 1,4-BDT can bridge two gold electrodes to form single-molecule junctions. [, , ] This property has made it a model system for studying electron transport at the single-molecule level. [, , ] The conductance of these junctions is sensitive to the molecule's configuration and its interaction with the electrodes. [, , ]
Q6: How is this compound used in the fabrication of hybrid solar cells?
A6: this compound can be employed as a ligand in hybrid solar cells incorporating poly(3-hexylthiophene) (P3HT) and lead sulfide (PbS) quantum dots (QDs). [] Replacing longer-chain ligands with 1,4-BDT through post-deposition ligand exchange significantly enhances the solar cell performance. [] This improvement is attributed to a reduced barrier for electron transfer and optimized phase separation between the P3HT and PbS QDs. []
Q7: What is the role of this compound in the synthesis of poly(benzothiazole)s?
A7: this compound is a key monomer in the synthesis of poly(benzothiazole)s. [, ] It can undergo polycondensation reactions with various dicarboxylic acids in the presence of a condensing agent like phosphorus pentoxide/methanesulfonic acid. [, ] The resulting polymers exhibit high molecular weights and excellent thermal stability, making them attractive for high-performance applications. [, ]
Q8: Can this compound be used to prepare polymers with anthracene units in the main chain?
A8: Yes, 1,4-BDT can polymerize with 9,10-diethynylanthracene to yield conjugated polymers containing anthracene units within the polymer backbone. [, ] The polymerization can be initiated through methods like UV irradiation or using initiators like AIBN or BPO. [, ] The polymer's cis/trans ratio is influenced by the initiation method and reaction conditions. [, ]
Q9: How has computational chemistry been employed to study this compound?
A9: Computational methods like Density Functional Theory (DFT) are instrumental in investigating various aspects of 1,4-BDT. [, , ] These include optimizing its geometry, calculating its Raman scattering spectra, understanding its adsorption on metal surfaces, and simulating its behavior in single-molecule junctions. [, , ]
Q10: How does the structure of this compound influence its conductivity in single-molecule junctions?
A10: The conductivity of 1,4-BDT in single-molecule junctions is strongly dependent on its conformation and interaction with the electrodes. [, , ] Factors like the tilt angle of the benzene ring relative to the electrode surface, the distance between the sulfur atoms and the gold atoms, and the electronic coupling between the molecule and the electrodes all play a role in determining the conductance. [, , ]
Avertissement et informations sur les produits de recherche in vitro
Veuillez noter que tous les articles et informations sur les produits présentés sur BenchChem sont destinés uniquement à des fins informatives. Les produits disponibles à l'achat sur BenchChem sont spécifiquement conçus pour des études in vitro, qui sont réalisées en dehors des organismes vivants. Les études in vitro, dérivées du terme latin "in verre", impliquent des expériences réalisées dans des environnements de laboratoire contrôlés à l'aide de cellules ou de tissus. Il est important de noter que ces produits ne sont pas classés comme médicaments et n'ont pas reçu l'approbation de la FDA pour la prévention, le traitement ou la guérison de toute condition médicale, affection ou maladie. Nous devons souligner que toute forme d'introduction corporelle de ces produits chez les humains ou les animaux est strictement interdite par la loi. Il est essentiel de respecter ces directives pour assurer la conformité aux normes légales et éthiques en matière de recherche et d'expérimentation.