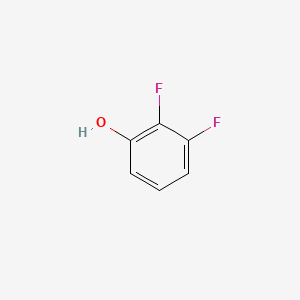
2,3-Difluorophenol
Vue d'ensemble
Description
2,3-Difluorophenol (CAS 6418-38-8) is a fluorinated aromatic compound with the molecular formula C₆H₄F₂O. Its structure features hydroxyl and fluorine substituents at the 1-, 2-, and 3-positions of the benzene ring. This compound is a key intermediate in organic synthesis, particularly in the development of hydrogen-bond acidic polymers for gas sensing applications . Its high acidity (attributed to electron-withdrawing fluorine atoms) and reactivity make it valuable in materials science and pharmaceutical chemistry.
Méthodes De Préparation
Grignard Reagent-Mediated Boronation and Oxidation
The most well-documented approach for synthesizing fluorophenols involves the sequential formation of Grignard reagents, boronation, and oxidative hydrolysis. While originally developed for 4-ethoxy-2,3-difluorophenol , this method is adaptable to 2,3-difluorophenol by modifying the starting material.
Reaction Mechanism and Stepwise Protocol
The process begins with 2,3-difluorobromobenzene as the starting material. Under nitrogen protection, magnesium reacts with iodine-initiated tetrahydrofuran (THF) to form a Grignard reagent. Subsequent boronation with trimethyl borate yields 2,3-difluorophenylboronic acid, which is oxidized to the target phenol using hydrogen peroxide .
Critical Parameters :
-
Grignard Formation : Optimal temperatures range from 0–60°C, with iodine enhancing initiation efficiency. Lower temperatures (−40°C to −20°C) during boronation minimize side reactions .
-
Oxidation : Hydrogen peroxide (30–50% concentration) at 10–40°C ensures complete conversion of the boronic acid to phenol without over-oxidation .
Yield and Purity :
-
Pilot-scale trials for analogous compounds report yields of 74–75% after purification, with HPLC purity exceeding 99.5% .
Nucleophilic Aromatic Substitution (SNAr)
Nucleophilic substitution offers an alternative route, particularly for introducing fluorine atoms at specific positions. This method typically requires electron-deficient aromatic rings activated toward nucleophilic attack.
Substrate Activation and Fluorination
Starting with 2,3-dichlorophenol , fluoride ions (e.g., KF or CsF) displace chlorine atoms under high-temperature conditions (150–200°C) in polar aprotic solvents like dimethylformamide (DMF). Catalysts such as 18-crown-6 improve fluoride solubility and reactivity.
Challenges :
-
Regioselectivity : Ensuring exclusive substitution at the 2- and 3-positions requires precise control of reaction conditions.
-
Byproducts : Competing hydrolysis or dehalogenation can reduce yields, necessitating excess fluoride and inert atmospheres.
Performance Metrics :
-
Limited literature data suggest modest yields (40–60%) due to competing side reactions, making this method less favorable for industrial use compared to Grignard pathways.
Hydrolysis of Protected Ethers
Hydrolytic cleavage of alkoxy- or aryloxy-protected precursors provides a straightforward route to phenols. For this compound, 2,3-difluoroanisole (methoxy-protected derivative) is a viable intermediate.
Demethylation Strategies
Lewis acids like boron tribromide (BBr₃) or aluminum chloride (AlCl₃) cleave methoxy groups at low temperatures (−78°C to 0°C). For example:
3, \text{CH}2\text{Cl}2, -20^\circ\text{C}} \text{this compound} + \text{CH}3\text{Br}
Advantages :
-
High regiochemical fidelity.
-
Compatible with sensitive functional groups.
Limitations :
-
Requires stoichiometric amounts of hazardous Lewis acids.
-
Post-reaction quenching generates significant waste.
Diazonium Salt Fluorination
Diazotization followed by fluorination (Balz-Schiemann reaction) is a classical method for introducing fluorine atoms. Starting with 2,3-diaminophenol , diazonium salts are generated and decomposed in the presence of fluoroboric acid (HBF₄).
Reaction Sequence
-
Diazotization : Treatment with NaNO₂ and HCl at 0–5°C forms the diazonium salt.
-
Fluorination : Thermal decomposition of the diazonium tetrafluoroborate at 100–120°C releases nitrogen gas and yields this compound.
Considerations :
-
Safety : Exothermic decomposition necessitates careful temperature control.
-
Yield : Reported yields range from 50–65%, with purity dependent on efficient salt isolation.
Direct Fluorination Techniques
Electrophilic fluorination using agents like Selectfluor® or acetyl hypofluorite (AcOF) enables direct C–H fluorination. However, achieving di-ortho fluorination on phenol rings remains challenging due to electronic and steric factors.
Directed Ortho-Metalation (DoM)
A directed metalation strategy employs phenol derivatives with directing groups (e.g., –OMe, –NMe₂). Lithiation at −78°C followed by fluorination with N-fluorobenzenesulfonimide (NFSI) installs fluorine atoms sequentially.
Example :
Efficiency :
-
Multi-step synthesis complicates scalability.
-
Yields rarely exceed 40% due to incomplete lithiation and competing side reactions.
Comparative Analysis of Synthetic Methods
Analyse Des Réactions Chimiques
2,3-Difluorophenol undergoes various chemical reactions, including:
Electrophilic Substitution: It can react with nitric acid in the presence of sulfuric acid to form 2,3-difluoro-6-nitrophenol.
Oxidation: It can be oxidized to form corresponding quinones under specific conditions.
Reduction: It can be reduced to form difluorocyclohexanol derivatives.
Substitution: It can undergo nucleophilic substitution reactions where the fluorine atoms are replaced by other nucleophiles.
Common reagents used in these reactions include nitric acid, sulfuric acid, n-butyllithium, and hydrogen peroxide. The major products formed depend on the specific reaction conditions and reagents used.
Applications De Recherche Scientifique
Synthesis and Reactivity
2,3-Difluorophenol can be synthesized through various methods, including direct fluorination of phenol derivatives. Its unique structure allows it to participate in several chemical reactions, including:
- Nucleophilic Substitution : The hydroxyl group can be substituted with other functional groups.
- Oxidation and Reduction Reactions : It can be oxidized to form aldehydes or carboxylic acids and reduced to other phenolic derivatives.
Scientific Research Applications
This compound has been utilized in multiple scientific domains:
Material Science
This compound is employed in the synthesis of advanced materials. For instance, it was used in creating poly{methyl[3-(2-hydroxy-3,4-difluoro)phenyl]propyl siloxane}, a material sensitive to toxic organophosphate vapors. This polymer exhibits potential applications in environmental monitoring and safety .
Biological Research
The compound is being studied for its biological activities:
- Antimicrobial Properties : Preliminary studies indicate that this compound exhibits antimicrobial activity against various pathogens.
- Enzyme Inhibition : It has been shown to inhibit certain enzymes, which could have implications for drug development.
Pharmaceutical Applications
Due to its ability to interact with biological targets, this compound is being investigated as a potential therapeutic agent. Its fluorinated structure enhances binding affinities, making it a candidate for developing new pharmaceuticals .
Table 1: Comparison of Fluorinated Phenols
Compound Name | Structure Features | Unique Aspects |
---|---|---|
This compound | Fluorine at positions 2 and 3 | Enhanced reactivity due to fluorination |
2,4-Difluorophenol | Fluorine at positions 2 and 4 | Different biological activity profiles |
3-Fluorophenol | Fluorine at position 3 | Less sterically hindered compared to difluoros |
4-Fluorophenol | Fluorine at position 4 | Exhibits distinct chemical properties |
Case Study 1: In Vitro Studies on Enzyme Inhibition
Research demonstrated that this compound effectively inhibits aromatase activity with an IC50 value comparable to other known inhibitors. This suggests potential applications in treating hormone-dependent cancers .
Case Study 2: Cell-Based Assays
In NIH-3T3 cell lines expressing specific mutations, the compound was screened for its potential as a cystic fibrosis transmembrane conductance regulator potentiator. Results indicated promising activity, warranting further investigation into therapeutic applications .
Case Study 3: Toxicological Assessments
Studies on the stability of this compound in physiological conditions revealed variations impacting its safety profile and therapeutic index. Understanding these factors is crucial for evaluating its potential use in clinical settings .
Mécanisme D'action
The mechanism of action of 2,3-Difluorophenol depends on its application. In biochemical assays, it can act as an inhibitor or activator of specific enzymes by interacting with their active sites. In pharmaceutical applications, it can interact with molecular targets such as receptors or enzymes, modulating their activity and leading to therapeutic effects .
Comparaison Avec Des Composés Similaires
Structural and Electronic Properties
Fluorophenols exhibit distinct electronic and steric properties depending on the positions of fluorine substituents. Below is a comparative analysis of 2,3-difluorophenol with other difluorophenol isomers:
Key Insights :
- Acidity: 2,6-Difluorophenol (pKa 7.12) is more acidic than phenol (pKa 9.81) due to strong electron-withdrawing effects from fluorine . This compound’s acidity is comparable but slightly lower due to reduced resonance stabilization.
- NMR Shifts: The 19F NMR chemical shift of this compound (-111.8 ppm) differs significantly from 3,4-difluorophenol (-108.2 ppm), reflecting distinct electronic environments .
Key Insights :
- This compound’s synthesis often employs Mitsunobu reactions or boronic acid intermediates , while 2,6-difluorophenol leverages halogenation strategies optimized for para-substitution .
Key Insights :
- This compound’s high hydrogen-bond acidity makes it ideal for gas-sensing polymers like PMFOS, which detect polar volatile compounds .
- 2,6-Difluorophenol’s bioisosteric properties enable its use in CNS-active drugs to enhance blood-brain barrier penetration .
Thermal and Chemical Stability
Key Insights :
- This compound-derived polymers (e.g., PMFOS) exhibit thermal stability suitable for sensor applications .
Activité Biologique
2,3-Difluorophenol (DFP) is a fluorinated aromatic compound that has garnered attention in medicinal chemistry and biochemistry due to its unique biological activities. This article reviews the biological activity of this compound, focusing on its pharmacological properties, mechanisms of action, and potential applications in drug development.
Molecular Formula : CHFO
Molecular Weight : 148.09 g/mol
Melting Point : 39-42 °C
Boiling Point : 54 °C at 25 mmHg
The presence of fluorine atoms significantly alters the physicochemical properties of phenolic compounds, enhancing their lipophilicity and metabolic stability, which can contribute to their biological activity .
This compound exhibits various biological activities primarily attributed to its ability to interact with biological macromolecules. Some key mechanisms include:
- Enzyme Inhibition : DFP has been shown to inhibit specific enzymes involved in metabolic pathways. For instance, it demonstrates inhibitory effects on glycoside hydrolases, which are crucial for carbohydrate metabolism .
- Antitumor Activity : Recent studies indicate that DFP and its derivatives can exhibit antitumor properties. The compound's structural modifications have been linked to enhanced cytotoxicity against cancer cell lines, such as MCF-7 (breast cancer) and others .
Table 1: Biological Activities of this compound
Case Study 1: Antitumor Effects
In a study examining the antitumor effects of various fluorinated compounds, including DFP, it was found that DFP exhibited significant cytotoxic effects on MCF-7 cells. The IC50 values indicated a dose-dependent response, with DFP showing enhanced potency compared to non-fluorinated analogs . This suggests that the fluorination at the 2 and 3 positions is critical for its biological efficacy.
Case Study 2: Enzyme Interaction
Another investigation focused on the interaction of DFP with glycoside hydrolases. The study revealed that DFP could bind covalently to the active site residues of these enzymes, leading to a decrease in enzymatic activity. This finding highlights the potential of DFP as a lead compound for developing enzyme inhibitors in therapeutic applications .
Q & A
Basic Research Questions
Q. What are the most reliable synthetic routes for 2,3-difluorophenol, and how can experimental conditions be optimized to improve yield?
- Methodological Answer : Current synthesis methods (e.g., diazotization-hydrolysis of 2,3,4-trifluoroaniline or H₂O₂-mediated oxidation of 2,3-difluorophenylboronic acid) often suffer from low yields (<50%) and multi-step complexity . To optimize, consider reaction parameter screening (temperature, solvent polarity, catalyst loading) and real-time monitoring via HPLC or GC-MS to identify bottlenecks. Evidence from high-yield (82%) protocols using phase-transfer catalysts (e.g., K₂CO₃ in acetone) suggests that controlled deprotonation and intermediate stabilization are critical .
Q. How can spectroscopic techniques (e.g., NMR, IR) confirm the structure and purity of this compound?
- Methodological Answer : ¹H and ¹³C NMR are essential for structural confirmation. For example, in ¹H NMR (CDCl₃), the hydroxyl proton appears as a broad singlet (~5 ppm), while fluorine substituents deshield adjacent protons, producing distinct splitting patterns (e.g., doublets of doublets). ¹⁹F NMR can further resolve positional isomers . Purity (>97%) is validated via HPLC with UV detection (230–265 nm) and comparison to commercial standards .
Q. What are the key physical properties (e.g., melting point, solubility) of this compound, and how are they experimentally determined?
- Methodological Answer : Reported melting points range from 34–36°C (pure) to 38–41°C (technical grade), determined via differential scanning calorimetry (DSC) . Solubility in polar solvents (e.g., acetone, ethanol) is assessed gravimetrically, while logP values (~1.8) are calculated via shake-flask or chromatographic methods .
Q. What safety protocols are critical when handling this compound in laboratory settings?
- Methodological Answer : Classified as a flammable liquid (GHS Category 3) and acute oral toxin (Category 4), it requires storage in flame-proof cabinets and handling under inert atmosphere. Use PPE (gloves, goggles) and emergency measures: neutralize spills with sand/diatomite, and rinse exposure sites with copious water .
Advanced Research Questions
Q. How do fluorination patterns (2,3-difluoro vs. 3,4-difluoro isomers) influence the compound’s reactivity in cross-coupling reactions?
- Methodological Answer : Fluorine’s electron-withdrawing effect activates the aromatic ring for nucleophilic substitution but deactivates it for electrophilic reactions. Computational studies (e.g., density functional theory, DFT) reveal that this compound’s LUMO energy is −1.8 eV, favoring SNAr reactions at the 4-position. Contrast this with 3,4-difluorophenol, where steric hindrance reduces reactivity .
Q. What contradictions exist in reported biological activities of this compound derivatives, and how can they be resolved?
- Methodological Answer : While some studies highlight its role as a virulence-suppressing pharmacophore in Staphylococcus aureus , others note negligible antimicrobial activity. To reconcile discrepancies, standardize bioassays (e.g., MIC testing in Mueller-Hinton broth) and control for derivative purity and stereochemistry. Conflicting structural data (e.g., misassigned substituent positions in early studies) must be verified via X-ray crystallography .
Q. How can synthetic byproducts (e.g., 2,3-difluoro-6-nitrophenol) be minimized during nitration reactions?
- Methodological Answer : Byproduct formation arises from competing meta-nitration due to fluorine’s directing effects. Optimize reaction conditions: use fuming HNO₃ at 0°C to favor para-nitration, or employ regioselective catalysts like zeolites. Monitor reaction progress with TLC (silica gel, hexane:EtOAc 4:1) .
Q. What computational tools predict the environmental toxicity of this compound, and how do they align with experimental data?
- Methodological Answer : QSAR models (e.g., ECOSAR) predict acute aquatic toxicity (LC₅₀ ~10 mg/L for fish), consistent with experimental OECD 203 testing . Discrepancies may arise from biodegradation pathways; use HPLC-MS to track metabolite formation (e.g., fluorocatechol) in simulated wastewater .
Propriétés
IUPAC Name |
2,3-difluorophenol | |
---|---|---|
Source | PubChem | |
URL | https://pubchem.ncbi.nlm.nih.gov | |
Description | Data deposited in or computed by PubChem | |
InChI |
InChI=1S/C6H4F2O/c7-4-2-1-3-5(9)6(4)8/h1-3,9H | |
Source | PubChem | |
URL | https://pubchem.ncbi.nlm.nih.gov | |
Description | Data deposited in or computed by PubChem | |
InChI Key |
RPEPGIOVXBBUMJ-UHFFFAOYSA-N | |
Source | PubChem | |
URL | https://pubchem.ncbi.nlm.nih.gov | |
Description | Data deposited in or computed by PubChem | |
Canonical SMILES |
C1=CC(=C(C(=C1)F)F)O | |
Source | PubChem | |
URL | https://pubchem.ncbi.nlm.nih.gov | |
Description | Data deposited in or computed by PubChem | |
Molecular Formula |
C6H4F2O | |
Source | PubChem | |
URL | https://pubchem.ncbi.nlm.nih.gov | |
Description | Data deposited in or computed by PubChem | |
DSSTOX Substance ID |
DTXSID40214381 | |
Record name | Phenol, 2,3-difluoro- | |
Source | EPA DSSTox | |
URL | https://comptox.epa.gov/dashboard/DTXSID40214381 | |
Description | DSSTox provides a high quality public chemistry resource for supporting improved predictive toxicology. | |
Molecular Weight |
130.09 g/mol | |
Source | PubChem | |
URL | https://pubchem.ncbi.nlm.nih.gov | |
Description | Data deposited in or computed by PubChem | |
CAS No. |
6418-38-8 | |
Record name | Phenol, 2,3-difluoro- | |
Source | ChemIDplus | |
URL | https://pubchem.ncbi.nlm.nih.gov/substance/?source=chemidplus&sourceid=0006418388 | |
Description | ChemIDplus is a free, web search system that provides access to the structure and nomenclature authority files used for the identification of chemical substances cited in National Library of Medicine (NLM) databases, including the TOXNET system. | |
Record name | Phenol, 2,3-difluoro- | |
Source | EPA DSSTox | |
URL | https://comptox.epa.gov/dashboard/DTXSID40214381 | |
Description | DSSTox provides a high quality public chemistry resource for supporting improved predictive toxicology. | |
Record name | 2,3-difluorophenol | |
Source | European Chemicals Agency (ECHA) | |
URL | https://echa.europa.eu/information-on-chemicals | |
Description | The European Chemicals Agency (ECHA) is an agency of the European Union which is the driving force among regulatory authorities in implementing the EU's groundbreaking chemicals legislation for the benefit of human health and the environment as well as for innovation and competitiveness. | |
Explanation | Use of the information, documents and data from the ECHA website is subject to the terms and conditions of this Legal Notice, and subject to other binding limitations provided for under applicable law, the information, documents and data made available on the ECHA website may be reproduced, distributed and/or used, totally or in part, for non-commercial purposes provided that ECHA is acknowledged as the source: "Source: European Chemicals Agency, http://echa.europa.eu/". Such acknowledgement must be included in each copy of the material. ECHA permits and encourages organisations and individuals to create links to the ECHA website under the following cumulative conditions: Links can only be made to webpages that provide a link to the Legal Notice page. | |
Synthesis routes and methods I
Procedure details
Synthesis routes and methods II
Procedure details
Synthesis routes and methods III
Procedure details
Retrosynthesis Analysis
AI-Powered Synthesis Planning: Our tool employs the Template_relevance Pistachio, Template_relevance Bkms_metabolic, Template_relevance Pistachio_ringbreaker, Template_relevance Reaxys, Template_relevance Reaxys_biocatalysis model, leveraging a vast database of chemical reactions to predict feasible synthetic routes.
One-Step Synthesis Focus: Specifically designed for one-step synthesis, it provides concise and direct routes for your target compounds, streamlining the synthesis process.
Accurate Predictions: Utilizing the extensive PISTACHIO, BKMS_METABOLIC, PISTACHIO_RINGBREAKER, REAXYS, REAXYS_BIOCATALYSIS database, our tool offers high-accuracy predictions, reflecting the latest in chemical research and data.
Strategy Settings
Precursor scoring | Relevance Heuristic |
---|---|
Min. plausibility | 0.01 |
Model | Template_relevance |
Template Set | Pistachio/Bkms_metabolic/Pistachio_ringbreaker/Reaxys/Reaxys_biocatalysis |
Top-N result to add to graph | 6 |
Feasible Synthetic Routes
Avertissement et informations sur les produits de recherche in vitro
Veuillez noter que tous les articles et informations sur les produits présentés sur BenchChem sont destinés uniquement à des fins informatives. Les produits disponibles à l'achat sur BenchChem sont spécifiquement conçus pour des études in vitro, qui sont réalisées en dehors des organismes vivants. Les études in vitro, dérivées du terme latin "in verre", impliquent des expériences réalisées dans des environnements de laboratoire contrôlés à l'aide de cellules ou de tissus. Il est important de noter que ces produits ne sont pas classés comme médicaments et n'ont pas reçu l'approbation de la FDA pour la prévention, le traitement ou la guérison de toute condition médicale, affection ou maladie. Nous devons souligner que toute forme d'introduction corporelle de ces produits chez les humains ou les animaux est strictement interdite par la loi. Il est essentiel de respecter ces directives pour assurer la conformité aux normes légales et éthiques en matière de recherche et d'expérimentation.