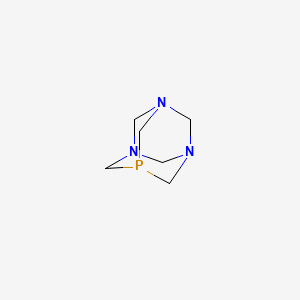
1,3,5-Triaza-7-phosphaadamantane
Vue d'ensemble
Description
1,3,5-Triaza-7-phosphaadamantane is a chemical compound with the formula C₆H₁₂N₃P. It is a product of the substitution of a nitrogen atom in hexamethylenetetramine with a phosphorus atom. This compound is known for its unique cage-like structure, which makes it highly stable and water-soluble. It is used as a ligand in transition metal complexes and as a catalyst in various organic reactions .
Applications De Recherche Scientifique
1,3,5-Triaza-7-phosphaadamantane has a wide range of scientific research applications:
Mécanisme D'action
Target of Action
1,3,5-Triaza-7-phosphaadamantane (PTA) is primarily used as a ligand for transition metal complexes . It is also used as a catalyst for Baylis–Hillman reactions . The compound has been shown to have superior antiproliferative activity when tested in 3D spheroids of human pancreatic cancer cells and showed a capacity to inhibit thioredoxin reductase (TrxR) .
Mode of Action
PTA interacts with its targets through coordination chemistry. It forms complexes with transition metals, acting as a ligand . In the case of Baylis–Hillman reactions, PTA acts as an organocatalyst . When the PTA phosphorus atom is coordinated to a metal, the range of change in the chemical shift exceeds 100 ppm .
Biochemical Pathways
It is known that pta can form luminescent silver–organic frameworks . These frameworks are generated from silver(I) oxide, PTA, and aromatic carboxylic acids . The topological analysis of these frameworks reveals complex 3D networks .
Pharmacokinetics
It is known that pta is soluble in water, methanol, trichloromethane, acetone, ethanol, and dmso, but insoluble in hydrocarbon solvents . This suggests that PTA may have good bioavailability due to its solubility in various solvents.
Result of Action
The result of PTA’s action depends on its application. As a ligand for transition metal complexes, PTA can facilitate various chemical reactions . As a catalyst for Baylis–Hillman reactions, PTA can help generate adducts from electrophiles and activated alkenes . In biological systems, PTA has shown antiproliferative activity against human pancreatic cancer cells .
Action Environment
The action of PTA can be influenced by environmental factors. For example, the formation of PTA-driven 3D metal–organic frameworks depends on the presence of silver(I) oxide and aromatic carboxylic acids . Additionally, the solubility of PTA in various solvents suggests that its action may be influenced by the solvent environment .
Orientations Futures
The synthesis of new water-soluble N-alkylated derivatives of 1,3,5-triaza-7-phosphaadamantane is presented . The new compounds were fully characterized and their catalytic activity was investigated for the low power microwave assisted one-pot azide–alkyne cycloaddition reaction in homogeneous aqueous medium to obtain disubstituted 1,2,3-triazoles .
Analyse Biochimique
Biochemical Properties
1,3,5-Triaza-7-phosphaadamantane plays a significant role in biochemical reactions, particularly as a ligand in transition metal complexes. It interacts with various enzymes, proteins, and other biomolecules. For instance, it forms complexes with ruthenium, which have shown potential in anticancer therapies . These complexes can inhibit enzymes like thioredoxin reductase, leading to a dysfunction in the intracellular redox state . Additionally, this compound interacts with proteins involved in cell signaling pathways, influencing various biochemical processes .
Cellular Effects
This compound has notable effects on different types of cells and cellular processes. In human colon carcinoma cells, gold complexes bearing this compound ligands have shown antiproliferative effects . These effects are likely due to the inhibition of thioredoxin reductase, causing a disruption in the intracellular redox state. Furthermore, these complexes can alter the cell cycle by activating p53 and inducing apoptosis through mitochondrial depolarization and the activation of caspase-3 . This compound also influences cell signaling pathways, gene expression, and cellular metabolism .
Molecular Mechanism
The molecular mechanism of this compound involves its interaction with biomolecules at the molecular level. It acts as a ligand, forming complexes with transition metals like ruthenium and gold . These complexes can inhibit enzymes such as thioredoxin reductase, leading to changes in the intracellular redox state . Additionally, this compound can influence gene expression by interacting with proteins involved in transcriptional regulation . The binding interactions with biomolecules and enzyme inhibition or activation are crucial for its biochemical effects .
Temporal Effects in Laboratory Settings
In laboratory settings, the effects of this compound can change over time. Its stability and degradation are important factors to consider. Studies have shown that this compound is relatively stable under standard laboratory conditions . Its long-term effects on cellular function can vary depending on the experimental conditions. In vitro and in vivo studies have demonstrated that this compound can maintain its activity over extended periods, but its effects may diminish with prolonged exposure .
Dosage Effects in Animal Models
The effects of this compound vary with different dosages in animal models. At lower doses, it has shown potential therapeutic effects, particularly in anticancer therapies . At higher doses, it can exhibit toxic or adverse effects. Studies have indicated that there is a threshold beyond which the compound’s beneficial effects are outweighed by its toxicity . It is crucial to determine the optimal dosage to maximize its therapeutic potential while minimizing adverse effects .
Metabolic Pathways
This compound is involved in various metabolic pathways. It interacts with enzymes and cofactors that play a role in its metabolism. For instance, it can form complexes with transition metals, influencing metabolic flux and metabolite levels . These interactions can affect the overall metabolic pathways in which this compound is involved . Understanding these pathways is essential for elucidating its biochemical effects .
Transport and Distribution
The transport and distribution of this compound within cells and tissues are critical for its activity. It can interact with transporters and binding proteins that facilitate its movement across cellular membranes . Additionally, its localization and accumulation within specific cellular compartments can influence its biochemical effects . Understanding the transport and distribution mechanisms is crucial for optimizing its therapeutic potential .
Subcellular Localization
This compound exhibits specific subcellular localization, which can affect its activity and function. It can be directed to specific compartments or organelles through targeting signals or post-translational modifications . This localization is essential for its interaction with biomolecules and its overall biochemical effects . Understanding the subcellular localization of this compound is crucial for elucidating its mechanism of action .
Méthodes De Préparation
1,3,5-Triaza-7-phosphaadamantane can be synthesized through several methods. One common synthetic route involves the reaction of hexamethylenetetramine with tetrakis(hydroxymethyl)phosphonium chloride, sodium hydroxide, and formaldehyde in water . This reaction yields this compound as the final product. Industrial production methods typically follow similar synthetic routes but on a larger scale, ensuring the purity and consistency of the compound.
Analyse Des Réactions Chimiques
1,3,5-Triaza-7-phosphaadamantane undergoes various chemical reactions, including:
Oxidation: The compound can be oxidized to form phosphine oxides.
Reduction: It can be reduced to form phosphines.
Substitution: The nitrogen and phosphorus atoms in the compound can participate in substitution reactions, forming various derivatives.
Coordination: It acts as a ligand, coordinating with transition metals to form complexes.
Common reagents used in these reactions include oxidizing agents like hydrogen peroxide, reducing agents like lithium aluminum hydride, and various metal salts for coordination reactions. The major products formed from these reactions depend on the specific reagents and conditions used.
Comparaison Avec Des Composés Similaires
1,3,5-Triaza-7-phosphaadamantane is unique due to its cage-like structure and high water solubility. Similar compounds include:
3,7-Dimethyl-1,3,7-triaza-5-phosphabicyclo[3.3.1]nonane: This compound has a similar structure but with additional methyl groups, affecting its reactivity and solubility.
Triphenylphosphine: A common phosphine ligand, but it lacks the cage-like structure and water solubility of this compound.
These comparisons highlight the unique properties of this compound, making it a valuable compound in various scientific and industrial applications.
Propriétés
IUPAC Name |
1,3,5-triaza-7-phosphatricyclo[3.3.1.13,7]decane | |
---|---|---|
Source | PubChem | |
URL | https://pubchem.ncbi.nlm.nih.gov | |
Description | Data deposited in or computed by PubChem | |
InChI |
InChI=1S/C6H12N3P/c1-7-2-9-3-8(1)5-10(4-7)6-9/h1-6H2 | |
Source | PubChem | |
URL | https://pubchem.ncbi.nlm.nih.gov | |
Description | Data deposited in or computed by PubChem | |
InChI Key |
FXXRPTKTLVHPAR-UHFFFAOYSA-N | |
Source | PubChem | |
URL | https://pubchem.ncbi.nlm.nih.gov | |
Description | Data deposited in or computed by PubChem | |
Canonical SMILES |
C1N2CN3CN1CP(C2)C3 | |
Source | PubChem | |
URL | https://pubchem.ncbi.nlm.nih.gov | |
Description | Data deposited in or computed by PubChem | |
Molecular Formula |
C6H12N3P | |
Source | PubChem | |
URL | https://pubchem.ncbi.nlm.nih.gov | |
Description | Data deposited in or computed by PubChem | |
DSSTOX Substance ID |
DTXSID40201812 | |
Record name | 1,3,5-Triaza-7-phosphaadamantane | |
Source | EPA DSSTox | |
URL | https://comptox.epa.gov/dashboard/DTXSID40201812 | |
Description | DSSTox provides a high quality public chemistry resource for supporting improved predictive toxicology. | |
Molecular Weight |
157.15 g/mol | |
Source | PubChem | |
URL | https://pubchem.ncbi.nlm.nih.gov | |
Description | Data deposited in or computed by PubChem | |
CAS No. |
53597-69-6 | |
Record name | 1,3,5-Triaza-7-phosphaadamantane | |
Source | CAS Common Chemistry | |
URL | https://commonchemistry.cas.org/detail?cas_rn=53597-69-6 | |
Description | CAS Common Chemistry is an open community resource for accessing chemical information. Nearly 500,000 chemical substances from CAS REGISTRY cover areas of community interest, including common and frequently regulated chemicals, and those relevant to high school and undergraduate chemistry classes. This chemical information, curated by our expert scientists, is provided in alignment with our mission as a division of the American Chemical Society. | |
Explanation | The data from CAS Common Chemistry is provided under a CC-BY-NC 4.0 license, unless otherwise stated. | |
Record name | 1,3,5-Triaza-7-phosphaadamantane | |
Source | ChemIDplus | |
URL | https://pubchem.ncbi.nlm.nih.gov/substance/?source=chemidplus&sourceid=0053597696 | |
Description | ChemIDplus is a free, web search system that provides access to the structure and nomenclature authority files used for the identification of chemical substances cited in National Library of Medicine (NLM) databases, including the TOXNET system. | |
Record name | 53597-69-6 | |
Source | DTP/NCI | |
URL | https://dtp.cancer.gov/dtpstandard/servlet/dwindex?searchtype=NSC&outputformat=html&searchlist=266642 | |
Description | The NCI Development Therapeutics Program (DTP) provides services and resources to the academic and private-sector research communities worldwide to facilitate the discovery and development of new cancer therapeutic agents. | |
Explanation | Unless otherwise indicated, all text within NCI products is free of copyright and may be reused without our permission. Credit the National Cancer Institute as the source. | |
Record name | 1,3,5-Triaza-7-phosphaadamantane | |
Source | EPA DSSTox | |
URL | https://comptox.epa.gov/dashboard/DTXSID40201812 | |
Description | DSSTox provides a high quality public chemistry resource for supporting improved predictive toxicology. | |
Record name | 1,3,5-Triaza-7-phosphaadamantane | |
Source | European Chemicals Agency (ECHA) | |
URL | https://echa.europa.eu/information-on-chemicals | |
Description | The European Chemicals Agency (ECHA) is an agency of the European Union which is the driving force among regulatory authorities in implementing the EU's groundbreaking chemicals legislation for the benefit of human health and the environment as well as for innovation and competitiveness. | |
Explanation | Use of the information, documents and data from the ECHA website is subject to the terms and conditions of this Legal Notice, and subject to other binding limitations provided for under applicable law, the information, documents and data made available on the ECHA website may be reproduced, distributed and/or used, totally or in part, for non-commercial purposes provided that ECHA is acknowledged as the source: "Source: European Chemicals Agency, http://echa.europa.eu/". Such acknowledgement must be included in each copy of the material. ECHA permits and encourages organisations and individuals to create links to the ECHA website under the following cumulative conditions: Links can only be made to webpages that provide a link to the Legal Notice page. | |
Record name | 1,3,5-TRIAZA-7-PHOSPHAADAMANTANE | |
Source | FDA Global Substance Registration System (GSRS) | |
URL | https://gsrs.ncats.nih.gov/ginas/app/beta/substances/6W8JQP73M4 | |
Description | The FDA Global Substance Registration System (GSRS) enables the efficient and accurate exchange of information on what substances are in regulated products. Instead of relying on names, which vary across regulatory domains, countries, and regions, the GSRS knowledge base makes it possible for substances to be defined by standardized, scientific descriptions. | |
Explanation | Unless otherwise noted, the contents of the FDA website (www.fda.gov), both text and graphics, are not copyrighted. They are in the public domain and may be republished, reprinted and otherwise used freely by anyone without the need to obtain permission from FDA. Credit to the U.S. Food and Drug Administration as the source is appreciated but not required. | |
Retrosynthesis Analysis
AI-Powered Synthesis Planning: Our tool employs the Template_relevance Pistachio, Template_relevance Bkms_metabolic, Template_relevance Pistachio_ringbreaker, Template_relevance Reaxys, Template_relevance Reaxys_biocatalysis model, leveraging a vast database of chemical reactions to predict feasible synthetic routes.
One-Step Synthesis Focus: Specifically designed for one-step synthesis, it provides concise and direct routes for your target compounds, streamlining the synthesis process.
Accurate Predictions: Utilizing the extensive PISTACHIO, BKMS_METABOLIC, PISTACHIO_RINGBREAKER, REAXYS, REAXYS_BIOCATALYSIS database, our tool offers high-accuracy predictions, reflecting the latest in chemical research and data.
Strategy Settings
Precursor scoring | Relevance Heuristic |
---|---|
Min. plausibility | 0.01 |
Model | Template_relevance |
Template Set | Pistachio/Bkms_metabolic/Pistachio_ringbreaker/Reaxys/Reaxys_biocatalysis |
Top-N result to add to graph | 6 |
Feasible Synthetic Routes
Q1: What is the molecular formula and weight of 1,3,5-Triaza-7-phosphaadamantane (PTA)?
A1: this compound has the molecular formula C6H12N3P and a molecular weight of 157.16 g/mol.
Q2: What spectroscopic techniques are commonly employed for characterizing PTA and its complexes?
A: Commonly used techniques include Infrared (IR) spectroscopy, Nuclear Magnetic Resonance (NMR) spectroscopy (1H, 13C, and 31P), and mass spectrometry (MS) techniques such as FAB-MS+ and ESI-MS. These methods provide valuable information about the structure, bonding, and composition of PTA derivatives. [, , , , , , ]
Q3: How does the 31P NMR chemical shift of PTA reflect its coordination behavior?
A: The rigid cage-like structure of PTA makes its 31P NMR chemical shift highly sensitive to changes in its chemical environment. When PTA coordinates to a metal through the phosphorus atom, the 31P NMR signal experiences a significant downfield shift, exceeding 100 ppm compared to free PTA. This characteristic shift serves as a diagnostic tool for confirming metal coordination to PTA. []
Q4: What are some notable catalytic applications of PTA-metal complexes?
A4: PTA-metal complexes have demonstrated promising catalytic activity in various reactions, including:
- Aqueous biphasic buta-1,3-diene oligomerization and telomerization: Homoleptic nickel(0) and palladium(0) complexes with PTA catalyze these reactions efficiently in water, showcasing PTA's utility in green chemistry. []
- Baeyer-Villiger oxidation of ketones: Oxorhenium complexes containing PTA act as selective catalysts for this reaction, utilizing aqueous H2O2 as the oxidant. []
- Aqueous-phase carbon dioxide hydrogenation: (Pentamethylcyclopentadienyl)iridium-PTA complexes, particularly [Cp*Ir(PTA)2Cl]Cl, catalyze CO2 and bicarbonate hydrogenation under mild conditions in the absence of amines or other additives. []
Q5: How does the structure of PTA contribute to its effectiveness as a ligand in catalysis?
A5: The unique structure of PTA offers several advantages in catalysis:
- Small cone angle (102°): Enables the coordination of multiple PTA ligands to a single metal center, potentially enhancing catalytic activity. []
- Electronic properties: PTA acts as a relatively strong electron-donating ligand, influencing the electronic properties of the metal center and consequently its catalytic activity. []
Q6: How is DFT used in studying PTA and its complexes?
A6: Density Functional Theory (DFT) calculations have been instrumental in elucidating various aspects of PTA chemistry, including:
- Rationalizing the preference for P-coordination over N-coordination in PTA-rhenium complexes. []
- Predicting 31P NMR chemical shifts of PTA-metal complexes to support structural assignments in solution. []
- Investigating the mechanism of H2 activation in water by half-sandwich ruthenium-PTA complexes. []
Q7: How do modifications to the PTA scaffold affect its coordination behavior and catalytic activity?
A: Structural modifications to both the lower and upper rims of the PTA cage significantly impact its coordination chemistry and catalytic applications. []
- N-Alkylation (Lower Rim Modification): Can alter solubility, influence the coordination mode of PTA (P- vs. N-coordination), and affect catalytic activity and selectivity. [, , ]
- C-6 Functionalization (Upper Rim Modification): Introduction of various functional groups at the C-6 position allows for fine-tuning of steric and electronic properties, impacting catalytic performance. [, ]
Q8: What biological activities have been reported for PTA-metal complexes?
A8: PTA-metal complexes have shown promising activities in several biological areas:
- Antimicrobial activity: Silver(I) coordination polymers incorporating PTA and substituted glutarate or malonate units exhibited significant activity against various Gram-negative and Gram-positive bacteria, as well as a yeast strain. []
- Antiproliferative activity: Several PTA-containing metal complexes demonstrated promising antiproliferative activity against various cancer cell lines, including human lung carcinoma (A549), epithelioid cervix carcinoma (HeLa), and human breast adenocarcinoma (MCF-7). [, , , ]
Q9: How do PTA-metal complexes interact with DNA?
A: Studies suggest that PTA-metal complexes can interact with DNA through different mechanisms, including intercalation between DNA base pairs. The specific mode of interaction and the resulting biological effects depend on the nature of the metal ion and other ligands present in the complex. [, ]
Q10: What is the role of HSA interactions in the biological activity of PTA-metal complexes?
A: Human Serum Albumin (HSA) is a major protein in blood plasma, and its interactions with drugs can influence their pharmacokinetic and pharmacodynamic properties. Studies have shown that certain PTA-metal complexes can bind to HSA, potentially impacting their distribution and availability in biological systems. [, ]
Q11: What is known about the toxicity of PTA and its complexes?
A11: While PTA and many of its complexes exhibit promising biological activities, comprehensive toxicity studies are essential to assess their safety profile. Researchers are actively investigating the potential toxicity and long-term effects of these compounds.
Q12: How are PTA and its complexes quantified in different matrices?
A12: Various analytical techniques are employed to quantify PTA and its complexes, including:
- Elemental analysis: Provides information about the elemental composition of the compound. [, ]
- NMR spectroscopy: Allows for the identification and quantification of PTA and its complexes in solution. [, ]
- Mass spectrometry: Used to determine molecular weight and identify characteristic fragments. [, ]
Q13: How are single-crystal X-ray diffraction studies utilized in PTA research?
A: Single-crystal X-ray diffraction plays a crucial role in elucidating the solid-state structures of PTA and its complexes. These studies provide detailed information about bond lengths, bond angles, and coordination geometries, contributing significantly to the understanding of structure-activity relationships. [, , , , , , , , , , , ]
Q14: What are some potential future research directions for PTA and its derivatives?
A14: Research in the field of PTA chemistry is ongoing and multifaceted, with numerous avenues for future exploration:
- Development of new PTA-based ligands: Designing new PTA derivatives with tailored steric and electronic properties to optimize catalytic activity, selectivity, and biological activity. []
- Expanding the scope of catalytic applications: Exploring the use of PTA-metal complexes in a wider range of catalytic reactions, including those relevant to energy and sustainability. [, ]
- Investigating the therapeutic potential of PTA-metal complexes: Conducting in-depth preclinical and clinical studies to evaluate the efficacy and safety of promising PTA-containing drug candidates. [, , , ]
- Developing sustainable synthetic methodologies: Exploring green chemistry approaches for the synthesis of PTA and its derivatives, minimizing environmental impact. [, ]
Avertissement et informations sur les produits de recherche in vitro
Veuillez noter que tous les articles et informations sur les produits présentés sur BenchChem sont destinés uniquement à des fins informatives. Les produits disponibles à l'achat sur BenchChem sont spécifiquement conçus pour des études in vitro, qui sont réalisées en dehors des organismes vivants. Les études in vitro, dérivées du terme latin "in verre", impliquent des expériences réalisées dans des environnements de laboratoire contrôlés à l'aide de cellules ou de tissus. Il est important de noter que ces produits ne sont pas classés comme médicaments et n'ont pas reçu l'approbation de la FDA pour la prévention, le traitement ou la guérison de toute condition médicale, affection ou maladie. Nous devons souligner que toute forme d'introduction corporelle de ces produits chez les humains ou les animaux est strictement interdite par la loi. Il est essentiel de respecter ces directives pour assurer la conformité aux normes légales et éthiques en matière de recherche et d'expérimentation.