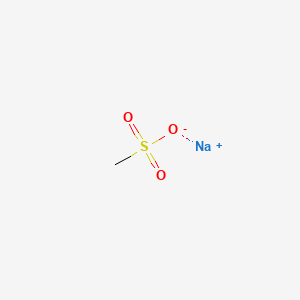
Méthanesulfonate de sodium
Vue d'ensemble
Description
Sodium methanesulfonate is a white crystalline powder characterized by its sodium and methanesulfonate functional groups. The molecular formula of sodium methanesulfonate is CH₃SO₃Na, and it has a molecular weight of 118.09 g/mol .
Applications De Recherche Scientifique
Sodium methanesulfonate has a wide range of applications in scientific research:
Chemistry: Used as a reagent for introducing methanesulfonate groups into organic molecules.
Biology: Employed in the synthesis of biologically active compounds.
Medicine: Utilized in the preparation of pharmaceutical intermediates.
Industry: Acts as a catalyst in various industrial processes, including esterification and transesterification.
Mécanisme D'action
Target of Action
Sodium methanesulfonate, also known as colistin methanesulfonate (CMS), is primarily used as a prodrug for colistin . Colistin targets multidrug-resistant Gram-negative bacteria, particularly Pseudomonas aeruginosa, Acinetobacter baumannii, and Klebsiella pneumoniae . It is also used as an anion source [CH3SO3]− for synthesizing ionic liquids .
Mode of Action
Sodium methanesulfonate is an inactive prodrug that is converted into its active form, colistin, in the body . Colistin interacts with the lipid A component of lipopolysaccharides in the outer membrane of Gram-negative bacteria, displacing divalent cations (Ca2+ and Mg2+) from the phosphate groups of membrane lipids. This leads to an increase in the permeability of the cell envelope, leakage of cellular contents, and ultimately cell death .
Biochemical Pathways
Pharmacokinetics
The pharmacokinetics of sodium methanesulfonate, when used as a prodrug for colistin, are complex . Due to its large molecular weight and cationic properties at physiological pH, colistin passes through physiological membranes poorly and is mainly distributed within the extracellular space . Renal clearance of colistin is very low, but the dosing regimen should be adapted to the renal function of the patient because CMS is partly eliminated by the kidney .
Result of Action
The primary result of sodium methanesulfonate’s action is the death of targeted Gram-negative bacteria. By increasing the permeability of the bacterial cell envelope, it causes leakage of cellular contents and eventually leads to cell death .
Action Environment
The action of sodium methanesulfonate can be influenced by various environmental factors. For instance, it is known that colistin binds to laboratory materials, which can raise many experimental issues and make studies on colistin tricky . Furthermore, resistance of bacteria to colistin is increasing worldwide in parallel to its clinical and veterinary uses . Therefore, the use of a loading dose might reduce the emergence of resistance but the use of colistin in combination also seems necessary .
Analyse Biochimique
Biochemical Properties
Sodium methanesulfonate plays a significant role in biochemical reactions, primarily as a source of methanesulfonate anions. It interacts with various enzymes and proteins, influencing their activity and stability. For instance, sodium methanesulfonate is used in the preparation of polyanionic antimicrobial membranes, where it interacts with microbial cell walls, disrupting their integrity and leading to cell lysis . Additionally, it is involved in the synthesis of indole acids, which act as inhibitors of phosphodiesterase enzymes, thereby modulating cellular signaling pathways .
Cellular Effects
Sodium methanesulfonate affects various types of cells and cellular processes. It has been observed to influence cell signaling pathways, gene expression, and cellular metabolism. For example, in microbial cells, sodium methanesulfonate disrupts cell wall integrity, leading to cell death . In mammalian cells, it can modulate enzyme activity, affecting metabolic pathways and gene expression. The compound’s impact on cell signaling pathways can lead to changes in cellular responses to external stimuli, thereby influencing overall cell function .
Molecular Mechanism
At the molecular level, sodium methanesulfonate exerts its effects through various mechanisms. It can bind to specific biomolecules, altering their structure and function. For instance, sodium methanesulfonate can inhibit enzyme activity by binding to the active site, preventing substrate binding and subsequent catalytic activity . Additionally, it can induce changes in gene expression by interacting with transcription factors or other regulatory proteins, leading to altered cellular responses .
Temporal Effects in Laboratory Settings
In laboratory settings, the effects of sodium methanesulfonate can change over time. The compound is relatively stable under standard storage conditions, but its activity can degrade over extended periods or under harsh conditions . Long-term exposure to sodium methanesulfonate in in vitro or in vivo studies has shown that it can lead to cumulative effects on cellular function, including changes in gene expression and metabolic activity . These temporal effects highlight the importance of considering the duration of exposure when designing experiments involving sodium methanesulfonate.
Dosage Effects in Animal Models
The effects of sodium methanesulfonate vary with different dosages in animal models. At low doses, the compound may have minimal impact on cellular function, while higher doses can lead to significant changes in cell signaling pathways, gene expression, and metabolic activity . In some cases, high doses of sodium methanesulfonate can cause toxic or adverse effects, such as cell death or tissue damage . These dosage-dependent effects underscore the importance of careful dose selection in experimental studies involving sodium methanesulfonate.
Metabolic Pathways
Sodium methanesulfonate is involved in various metabolic pathways, including those related to sulfur metabolism. It can be metabolized by bacteria and other microorganisms, leading to the production of methanesulfonate and other sulfur-containing compounds . The compound interacts with enzymes involved in sulfur metabolism, influencing metabolic flux and metabolite levels. These interactions can have downstream effects on cellular function and overall metabolic activity .
Transport and Distribution
Within cells and tissues, sodium methanesulfonate is transported and distributed through various mechanisms. It can interact with specific transporters or binding proteins, facilitating its movement across cellular membranes . Once inside the cell, sodium methanesulfonate can accumulate in specific compartments or organelles, influencing its localization and activity. These transport and distribution mechanisms are crucial for understanding the compound’s effects on cellular function .
Subcellular Localization
The subcellular localization of sodium methanesulfonate can affect its activity and function. The compound may be directed to specific compartments or organelles through targeting signals or post-translational modifications . For example, sodium methanesulfonate may localize to the cytoplasm, nucleus, or other organelles, where it can interact with specific biomolecules and influence cellular processes
Méthodes De Préparation
Synthetic Routes and Reaction Conditions: Sodium methanesulfonate can be synthesized by neutralizing methanesulfonic acid with sodium hydroxide. The reaction is typically carried out in an aqueous medium, and the resulting solution is evaporated to yield the crystalline product .
Industrial Production Methods: Industrial production of sodium methanesulfonate often involves the oxidation of dimethylsulfide using chlorine, followed by neutralization with sodium hydroxide. This method is efficient and yields high-purity sodium methanesulfonate .
Analyse Des Réactions Chimiques
Types of Reactions: Sodium methanesulfonate undergoes various chemical reactions, including:
Esterification and Transesterification: It acts as a catalyst in these reactions, facilitating the formation of esters from alcohols and acids.
Substitution Reactions: Sodium methanesulfonate can participate in nucleophilic substitution reactions, where the methanesulfonate group is replaced by other nucleophiles.
Common Reagents and Conditions:
Esterification: Typically involves alcohols and acids in the presence of sodium methanesulfonate as a catalyst.
Substitution: Common reagents include alkyl halides and nucleophiles such as amines or thiols.
Major Products:
Esterification: Produces esters and water.
Substitution: Produces substituted products where the methanesulfonate group is replaced by the nucleophile.
Comparaison Avec Des Composés Similaires
Methanesulfonic Acid: A closely related compound with similar chemical properties but is a liquid at room temperature.
Sodium p-toluenesulfonate: Another sulfonate salt used in similar applications but has a different aromatic structure.
Uniqueness: Sodium methanesulfonate is unique due to its high solubility in water and its ability to act as a versatile reagent in organic synthesis. Its crystalline form makes it easier to handle and store compared to its liquid counterpart, methanesulfonic acid .
Activité Biologique
Sodium methanesulfonate (CH₃SO₃Na) is a sulfonic acid salt that has garnered attention for its various biological activities, particularly in microbial metabolism and its role in the global sulfur cycle. This article delves into the biological significance of sodium methanesulfonate, highlighting its catabolism by microorganisms, potential applications in environmental science, and implications for human health.
Overview of Sodium Methanesulfonate
Sodium methanesulfonate is a stable compound that serves as a source of sulfur and carbon for various microbial communities. It is produced through the oxidation of dimethyl sulfide (DMS), which is a significant component of the biogeochemical sulfur cycle. Approximately 10^10 kg/year of methanesulfonate is estimated to be formed from DMS oxidation, making it an important intermediate in sulfur metabolism .
Microbial Catabolism
Microbial Utilization
Research has shown that sodium methanesulfonate can be utilized by specific bacteria as a carbon and energy source. The key enzyme involved in this process is methanesulfonate monooxygenase (MSAMO), which catalyzes the first step in the oxidation of methanesulfonate to formaldehyde, releasing sulfite as a byproduct. This reaction is crucial for the assimilation of methanesulfonate into microbial metabolic pathways .
Key Findings
- Enzymatic Activity : MSAMO has been identified in various marine bacteria, indicating that methanesulfonate catabolism is widespread among oceanic microbial populations.
- Environmental Impact : The catabolism of methanesulfonate contributes to cloud condensation nuclei (CCN) formation, influencing cloud properties and climate regulation .
Case Studies
-
Marine Bacterial Communities
A study investigated the presence and activity of msm genes responsible for methanesulfonate catabolism in marine environments. The findings revealed that these genes are actively expressed, suggesting that methanesulfonate plays a vital role in marine sulfur cycling and energy flow within microbial communities . -
Impact on Aerosol Formation
Another study utilized global aerosol models to assess the impact of methanesulfonate on aerosol size distributions. The results indicated that including methanesulfonate alters submicron aerosol mass and can influence climate through changes in cloud albedo .
Toxicological Aspects
Sodium methanesulfonate has been studied for its safety profile, particularly in pharmaceutical applications. It is important to note that while it serves as a prodrug for colistin (colistin methanesulfonate), it has limited antibacterial activity on its own. Colistin is activated from its prodrug form in vivo, showcasing sodium methanesulfonate's role as a carrier rather than an active therapeutic agent .
Comparative Biological Activity Table
Compound | Biological Activity | Key Findings |
---|---|---|
Sodium Methanesulfonate | Carbon and energy source for bacteria | Utilized by marine bacteria; significant in sulfur cycling |
Methanesulfonic Acid (MSA) | Influences aerosol formation | Alters cloud properties; impacts climate regulation |
Colistin Methanesulfonate | Prodrug form; limited activity | Requires conversion to colistin for antibacterial effects |
Propriétés
Numéro CAS |
2386-57-4 |
---|---|
Formule moléculaire |
CH4NaO3S |
Poids moléculaire |
119.10 g/mol |
Nom IUPAC |
sodium;methanesulfonate |
InChI |
InChI=1S/CH4O3S.Na/c1-5(2,3)4;/h1H3,(H,2,3,4); |
Clé InChI |
DWNWZFAKFWMPTI-UHFFFAOYSA-N |
SMILES |
CS(=O)(=O)[O-].[Na+] |
SMILES canonique |
CS(=O)(=O)O.[Na] |
Key on ui other cas no. |
2386-57-4 |
Pictogrammes |
Irritant |
Numéros CAS associés |
75-75-2 (Parent) |
Synonymes |
arium methanesulfonate BMS-480188 methanesulfonate methanesulfonic acid methanesulfonic acid, ammonia salt methanesulfonic acid, chromium (2+) salt methanesulfonic acid, chromium (3+) salt methanesulfonic acid, cobalt (2+) salt methanesulfonic acid, copper (2+) salt methanesulfonic acid, iron (2+) salt methanesulfonic acid, iron (3+)salt methanesulfonic acid, nickel (2+) salt methanesulfonic acid, potassium salt methanesulfonic acid, silver (1+) salt methanesulfonic acid, sodium salt methylsulfonate potassium mesylate potassium methanesulfonate |
Origine du produit |
United States |
Retrosynthesis Analysis
AI-Powered Synthesis Planning: Our tool employs the Template_relevance Pistachio, Template_relevance Bkms_metabolic, Template_relevance Pistachio_ringbreaker, Template_relevance Reaxys, Template_relevance Reaxys_biocatalysis model, leveraging a vast database of chemical reactions to predict feasible synthetic routes.
One-Step Synthesis Focus: Specifically designed for one-step synthesis, it provides concise and direct routes for your target compounds, streamlining the synthesis process.
Accurate Predictions: Utilizing the extensive PISTACHIO, BKMS_METABOLIC, PISTACHIO_RINGBREAKER, REAXYS, REAXYS_BIOCATALYSIS database, our tool offers high-accuracy predictions, reflecting the latest in chemical research and data.
Strategy Settings
Precursor scoring | Relevance Heuristic |
---|---|
Min. plausibility | 0.01 |
Model | Template_relevance |
Template Set | Pistachio/Bkms_metabolic/Pistachio_ringbreaker/Reaxys/Reaxys_biocatalysis |
Top-N result to add to graph | 6 |
Feasible Synthetic Routes
Q1: What is the molecular formula and weight of sodium methanesulfonate?
A1: Sodium methanesulfonate has the molecular formula CH3SO3Na and a molecular weight of 118.09 g/mol.
Q2: Are there any spectroscopic techniques used to characterize sodium methanesulfonate?
A: Yes, infrared (IR) spectroscopy has been used to study the state of molecular-ion equilibria of sodium methanesulfonate solutions in both water and methanesulfonic acid. []
Q3: How does the presence of sodium methanesulfonate affect the properties of sodium chloride (NaCl) particles?
A: Research indicates that sodium methanesulfonate can significantly influence both the hygroscopic and reactive properties of NaCl particles. Notably, the presence of sodium methanesulfonate leads to a decrease in the net reaction probability of the particle-HNO3 heterogeneous reaction. This effect is more pronounced at lower relative humidity levels and with increasing concentrations of sodium methanesulfonate. []
Q4: What unique structural characteristic of sodium methanesulfonate influences its interaction with sodium chloride particles?
A: The surfactant properties of methanesulfonate ions (CH3SO3-) contribute to their accumulation at the surface of wet, deliquesced NaCl particles. This surface accumulation plays a crucial role in altering the hygroscopic and reactive properties of the mixed particles. []
Q5: How does the presence of sodium methanesulfonate impact the deliquescence behavior of NaCl particles?
A: The deliquescence of mixed sodium methanesulfonate/NaCl particles occurs in two distinct steps. Initially, at approximately 69% relative humidity, the sodium methanesulfonate shell absorbs water. Subsequently, at around 75% relative humidity, the NaCl core undergoes deliquescence. This two-step deliquescence behavior differs from that of pure NaCl particles and highlights the influence of sodium methanesulfonate on the hygroscopic properties of the mixture. []
Q6: What is a notable application of sodium methanesulfonate in organic synthesis?
A: Sodium methanesulfonate serves as a valuable reagent in the synthesis of water-soluble 1,3,4-trisubstituted tetrahydropyrimidin-2(1H)-one derivatives. These derivatives, characterized by the presence of a sodium methanesulfonate fragment at the first position of the heterocycle, are prepared through a regioselective method involving the reaction of (1-(3,3-diethoxypropyl)ureido)methanesulfonate with various aromatic and heterocyclic nucleophiles. []
Q7: What are the advantages of using sodium methanesulfonate in the preparation of methanesulfonic acid compared to traditional methods?
A: Employing sodium methanesulfonate as a raw material in the production of methanesulfonic acid, particularly through bipolar membrane electrodialysis technology, offers several advantages over conventional organic synthesis methods. These include lower raw material costs, the ability to recycle alkali liquor (reducing preparation costs), avoidance of secondary environmental pollution, and an improved safety profile. []
Q8: How can the local head group concentration within sodium dodecyl sulfate and sodium dodecanesulfonate micelles be determined?
A: The local head group concentration in these micelles can be determined using chemical trapping. This involves employing 4-n-hexadecyl-2,6-dimethylbenzenediazonium tetrafluoroborate (16-ArN2BF4) as a probe and comparing the relative product yields obtained in the micellar solutions to those obtained in water using analogous short-chain compounds. []
Avertissement et informations sur les produits de recherche in vitro
Veuillez noter que tous les articles et informations sur les produits présentés sur BenchChem sont destinés uniquement à des fins informatives. Les produits disponibles à l'achat sur BenchChem sont spécifiquement conçus pour des études in vitro, qui sont réalisées en dehors des organismes vivants. Les études in vitro, dérivées du terme latin "in verre", impliquent des expériences réalisées dans des environnements de laboratoire contrôlés à l'aide de cellules ou de tissus. Il est important de noter que ces produits ne sont pas classés comme médicaments et n'ont pas reçu l'approbation de la FDA pour la prévention, le traitement ou la guérison de toute condition médicale, affection ou maladie. Nous devons souligner que toute forme d'introduction corporelle de ces produits chez les humains ou les animaux est strictement interdite par la loi. Il est essentiel de respecter ces directives pour assurer la conformité aux normes légales et éthiques en matière de recherche et d'expérimentation.