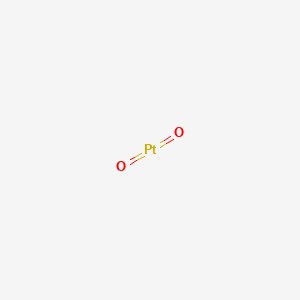
Oxyde de platine(IV)
Vue d'ensemble
Description
Platinum dioxide, also known as platinum(IV) oxide, is a chemical compound with the formula PtO₂. It is a dark brown powder that is commercially available and is often used as a catalyst in various chemical reactions. Platinum dioxide is particularly known for its role in hydrogenation and hydrogenolysis reactions in organic synthesis .
Applications De Recherche Scientifique
Platinum dioxide is widely used in scientific research due to its catalytic properties. Some of its applications include:
Chemistry: Used as a catalyst in organic synthesis for hydrogenation and hydrogenolysis reactions.
Biology: Employed in the study of enzyme-catalyzed reactions and metabolic pathways.
Medicine: Investigated for its potential use in drug synthesis and pharmaceutical applications.
Mécanisme D'action
Target of Action
Platinum(IV) oxide, also known as Platinum dioxide or Adams’ catalyst, primarily targets various functional groups in organic synthesis . It is used as a catalyst for hydrogenation and hydrogenolysis of these functional groups .
Mode of Action
It becomes active after exposure to hydrogen, whereupon it converts to platinum black, which is responsible for reactions . In polymer electrolyte fuel cells, the platinum catalyst in its active form is found predominantly in an oxidized state . Formation and reduction of surface oxide species determine both the electrocatalytic activity of the oxygen reduction reaction as well as the rate of corrosive Pt dissolution .
Biochemical Pathways
The biochemical pathways affected by Platinum(IV) oxide are complex and involve a range of surface processes. For instance, in polymer electrolyte fuel cells, the formation and reduction of surface oxide species are crucial . These processes are essential for both performance and durability of the fuel cells .
Pharmacokinetics
These drugs are associated with severe side effects, and an increasing number of drug-resistant tumors are reported in the clinics, limiting their clinical use .
Result of Action
The result of Platinum(IV) oxide’s action is primarily seen in its role as a catalyst. It facilitates hydrogenation and hydrogenolysis of various functional groups . In fuel cells, the formation and reduction of surface oxide species determine the electrocatalytic activity of the oxygen reduction reaction .
Action Environment
The action of Platinum(IV) oxide is influenced by environmental factors. For instance, the oxide becomes an active catalyst after exposure to hydrogen . Moreover, the formation and reduction of surface oxide species, which are crucial for the compound’s action, occur within a specific voltage range .
Analyse Biochimique
Biochemical Properties
Platinum(IV) oxide has been shown to interact with various biomolecules. It serves as a catalyst, effectively promoting the oxidation of hydrocarbons . The Platinum(IV) oxide interacts with these biomolecules, facilitating biochemical reactions.
Cellular Effects
Platinum(IV) oxide has been found to have significant effects on various types of cells. For instance, it has been shown to inhibit the proliferation and metastasis of cancer cells . It influences cell function by modulating cellular metabolism and impacting cell signaling pathways .
Molecular Mechanism
The molecular mechanism of Platinum(IV) oxide involves its conversion to platinum black after exposure to hydrogen, which is responsible for its reactivity . It exerts its effects at the molecular level through binding interactions with biomolecules, enzyme inhibition or activation, and changes in gene expression .
Temporal Effects in Laboratory Settings
The effects of Platinum(IV) oxide change over time in laboratory settings. It has been observed that the oxide becomes active and converts to platinum black after exposure to hydrogen . This indicates that the product’s stability and long-term effects on cellular function can vary depending on the conditions of the experiment .
Dosage Effects in Animal Models
The effects of Platinum(IV) oxide vary with different dosages in animal models. Studies have shown that platinum-based drugs can cause various adverse reactions in the human body through different routes of administration, including reproductive toxicity, genetic toxicity, and embryonic developmental toxicity .
Metabolic Pathways
Platinum(IV) oxide is involved in various metabolic pathways. It serves as a catalyst for hydrogenation and hydrogenolysis of various functional groups . It interacts with enzymes and cofactors in these pathways, potentially affecting metabolic flux or metabolite levels .
Méthodes De Préparation
Synthetic Routes and Reaction Conditions: Platinum dioxide is typically prepared from chloroplatinic acid (H₂PtCl₆) or ammonium chloroplatinate ((NH₄)₂PtCl₆) by fusion with sodium nitrate (NaNO₃). The process involves the following steps :
Preparation of Platinum Nitrate:
Formation of Platinum Dioxide:
The resulting brown cake is washed with water to remove nitrates and can be used as is or dried and stored for later use .
Industrial Production Methods: An alternative method involves a liquid phase process where a chloroplatinic acid water solution is prepared and its pH is regulated using a carbonate saturated water solution. The reaction is carried out at 70-90°C, followed by the addition of glacial acetic acid, filtration, washing, drying, and roasting to obtain platinum dioxide . This method is environmentally friendly and suitable for large-scale production.
Analyse Des Réactions Chimiques
Types of Reactions: Platinum dioxide undergoes various types of reactions, including:
Hydrogenation: Reduction of alkenes, ketones, and nitro compounds to corresponding alkanes, alcohols, and amines.
Hydrogenolysis: Cleavage of carbon-oxygen and carbon-nitrogen bonds.
Oxidation: Oxidation of carbon monoxide to carbon dioxide.
Common Reagents and Conditions:
Hydrogenation: Typically carried out in the presence of hydrogen gas.
Hydrogenolysis: Often performed with hydrogen gas under mild conditions.
Oxidation: Conducted in the presence of oxygen or air.
Major Products:
Hydrogenation: Alkanes, alcohols, and amines.
Hydrogenolysis: Deprotected alcohols and amines.
Oxidation: Carbon dioxide.
Comparaison Avec Des Composés Similaires
- Palladium oxide (PdO)
- Rhodium oxide (Rh₂O₃)
- Iridium oxide (IrO₂)
Platinum dioxide stands out due to its unique combination of stability, catalytic activity, and versatility in various chemical reactions.
Propriétés
IUPAC Name |
dioxoplatinum | |
---|---|---|
Source | PubChem | |
URL | https://pubchem.ncbi.nlm.nih.gov | |
Description | Data deposited in or computed by PubChem | |
InChI |
InChI=1S/2O.Pt | |
Source | PubChem | |
URL | https://pubchem.ncbi.nlm.nih.gov | |
Description | Data deposited in or computed by PubChem | |
InChI Key |
YKIOKAURTKXMSB-UHFFFAOYSA-N | |
Source | PubChem | |
URL | https://pubchem.ncbi.nlm.nih.gov | |
Description | Data deposited in or computed by PubChem | |
Canonical SMILES |
O=[Pt]=O | |
Source | PubChem | |
URL | https://pubchem.ncbi.nlm.nih.gov | |
Description | Data deposited in or computed by PubChem | |
Molecular Formula |
O2Pt | |
Source | PubChem | |
URL | https://pubchem.ncbi.nlm.nih.gov | |
Description | Data deposited in or computed by PubChem | |
DSSTOX Substance ID |
DTXSID40904275 | |
Record name | Platinic oxide | |
Source | EPA DSSTox | |
URL | https://comptox.epa.gov/dashboard/DTXSID40904275 | |
Description | DSSTox provides a high quality public chemistry resource for supporting improved predictive toxicology. | |
Molecular Weight |
227.08 g/mol | |
Source | PubChem | |
URL | https://pubchem.ncbi.nlm.nih.gov | |
Description | Data deposited in or computed by PubChem | |
Physical Description |
Other Solid, Monohydrate: Black solid; [Merck Index] Black odorless powder; Insoluble in water; [MSDSonline] | |
Record name | Platinum oxide (PtO2) | |
Source | EPA Chemicals under the TSCA | |
URL | https://www.epa.gov/chemicals-under-tsca | |
Description | EPA Chemicals under the Toxic Substances Control Act (TSCA) collection contains information on chemicals and their regulations under TSCA, including non-confidential content from the TSCA Chemical Substance Inventory and Chemical Data Reporting. | |
Record name | Platinic oxide | |
Source | Haz-Map, Information on Hazardous Chemicals and Occupational Diseases | |
URL | https://haz-map.com/Agents/8669 | |
Description | Haz-Map® is an occupational health database designed for health and safety professionals and for consumers seeking information about the adverse effects of workplace exposures to chemical and biological agents. | |
Explanation | Copyright (c) 2022 Haz-Map(R). All rights reserved. Unless otherwise indicated, all materials from Haz-Map are copyrighted by Haz-Map(R). No part of these materials, either text or image may be used for any purpose other than for personal use. Therefore, reproduction, modification, storage in a retrieval system or retransmission, in any form or by any means, electronic, mechanical or otherwise, for reasons other than personal use, is strictly prohibited without prior written permission. | |
CAS No. |
1314-15-4 | |
Record name | Platinum dioxide | |
Source | CAS Common Chemistry | |
URL | https://commonchemistry.cas.org/detail?cas_rn=1314-15-4 | |
Description | CAS Common Chemistry is an open community resource for accessing chemical information. Nearly 500,000 chemical substances from CAS REGISTRY cover areas of community interest, including common and frequently regulated chemicals, and those relevant to high school and undergraduate chemistry classes. This chemical information, curated by our expert scientists, is provided in alignment with our mission as a division of the American Chemical Society. | |
Explanation | The data from CAS Common Chemistry is provided under a CC-BY-NC 4.0 license, unless otherwise stated. | |
Record name | Platinic oxide | |
Source | ChemIDplus | |
URL | https://pubchem.ncbi.nlm.nih.gov/substance/?source=chemidplus&sourceid=0001314154 | |
Description | ChemIDplus is a free, web search system that provides access to the structure and nomenclature authority files used for the identification of chemical substances cited in National Library of Medicine (NLM) databases, including the TOXNET system. | |
Record name | Platinum dioxide | |
Source | DTP/NCI | |
URL | https://dtp.cancer.gov/dtpstandard/servlet/dwindex?searchtype=NSC&outputformat=html&searchlist=402624 | |
Description | The NCI Development Therapeutics Program (DTP) provides services and resources to the academic and private-sector research communities worldwide to facilitate the discovery and development of new cancer therapeutic agents. | |
Explanation | Unless otherwise indicated, all text within NCI products is free of copyright and may be reused without our permission. Credit the National Cancer Institute as the source. | |
Record name | Platinum oxide (PtO2) | |
Source | EPA Chemicals under the TSCA | |
URL | https://www.epa.gov/chemicals-under-tsca | |
Description | EPA Chemicals under the Toxic Substances Control Act (TSCA) collection contains information on chemicals and their regulations under TSCA, including non-confidential content from the TSCA Chemical Substance Inventory and Chemical Data Reporting. | |
Record name | Platinic oxide | |
Source | EPA DSSTox | |
URL | https://comptox.epa.gov/dashboard/DTXSID40904275 | |
Description | DSSTox provides a high quality public chemistry resource for supporting improved predictive toxicology. | |
Record name | Platinum dioxide | |
Source | European Chemicals Agency (ECHA) | |
URL | https://echa.europa.eu/substance-information/-/substanceinfo/100.013.840 | |
Description | The European Chemicals Agency (ECHA) is an agency of the European Union which is the driving force among regulatory authorities in implementing the EU's groundbreaking chemicals legislation for the benefit of human health and the environment as well as for innovation and competitiveness. | |
Explanation | Use of the information, documents and data from the ECHA website is subject to the terms and conditions of this Legal Notice, and subject to other binding limitations provided for under applicable law, the information, documents and data made available on the ECHA website may be reproduced, distributed and/or used, totally or in part, for non-commercial purposes provided that ECHA is acknowledged as the source: "Source: European Chemicals Agency, http://echa.europa.eu/". Such acknowledgement must be included in each copy of the material. ECHA permits and encourages organisations and individuals to create links to the ECHA website under the following cumulative conditions: Links can only be made to webpages that provide a link to the Legal Notice page. | |
Q1: What is the molecular formula and weight of platinum dioxide?
A1: Platinum dioxide has the molecular formula PtO2 and a molecular weight of 227.08 g/mol.
Q2: Are there different crystalline structures of platinum dioxide?
A2: Yes, platinum dioxide exists in three nearly energy-degenerate crystal structures: α-(CdI2), β-(CaCl2), and β'(rutile). [] At low temperatures and zero pressure, the α-phase is the most thermodynamically stable. []
Q3: Is platinum dioxide stable in air at room temperature?
A3: Yes, platinum dioxide, specifically the α-PtO2 phase (also known as Adam's catalyst), is relatively stable in air at room temperature. [, ]
Q4: Can platinum dioxide be reduced to metallic platinum?
A4: Yes, platinum dioxide can be readily reduced to metallic platinum using various methods. These include heating above 500°C, exposure to reducing agents like carbon monoxide or hydrogen, and even at room temperature with hydrogen. [, , ]
Q5: What happens to thin films of platinum dioxide upon heating in a vacuum?
A5: Thin films of platinum dioxide, when annealed at 720 K in a vacuum, experience the desorption of the oxide layer, ultimately recovering the platinum film. []
Q6: What are the main catalytic applications of platinum dioxide?
A6: Platinum dioxide, particularly in its α-PtO2 form (Adam's catalyst), is extensively used as a catalyst for hydrogenation reactions. [, , ] It has also been utilized in oxidation and reduction reactions. []
Q7: Does the size of platinum particles influence its catalytic oxidation?
A7: Yes, the particle size of dispersed platinum significantly impacts its oxidation behavior with dioxygen. Studies have shown that different particle sizes lead to variations in the extent of oxidation, the heat of oxidation, and even the types of surface platinum oxides formed. []
Q8: Can platinum dioxide catalyze reactions other than hydrogenation?
A8: Yes, platinum dioxide can mediate transesterification reactions under essentially neutral conditions. This catalytic activity has proven effective for various aromatic and aliphatic starting materials, achieving good to excellent yields. []
Q9: Is there a way to regenerate platinum from used platinum dioxide catalysts?
A9: Yes, platinum dioxide can be reduced to metallic platinum, which can then be recovered and potentially reused in new catalysts. [, ]
Q10: How does carbon presence affect platinum dioxide's properties?
A10: Hydrocarbons can chemisorb onto α-PtO2, lowering its decomposition temperature. This decomposition yields carbon dioxide and metallic platinum without forming intermediate platinum oxidation species. []
Q11: Have computational methods been used to study platinum dioxide?
A11: Yes, density functional theory (DFT) calculations have been employed to investigate the electronic properties of β-PtO2. These studies revealed that oxygen vacancies in the material can induce local magnetic moments on neighboring platinum and oxygen atoms. []
Q12: Is the α,β-unsaturated double bond in chalcone structures important for anticancer activity?
A12: Research suggests that the α,β-unsaturated double bond in chalcone structures plays a crucial role in their anticancer activity. This conclusion stems from the observation that the anticancer potency of Davidigenin (dihydro-isoliquiritigenin), where the double bond is reduced, is lower than its parent compound, isoliquiritigenin. []
Q13: What is the role of trehalose in formulating biosensors with platinum dioxide?
A13: Trehalose acts as a stabilizing agent for the enzyme alcohol dehydrogenase during the electropolymerization process with m-phenylenediamine. This stabilization is crucial for developing a functional biosensor that utilizes alcohol dehydrogenase and platinum dioxide for ethanol detection. []
Q14: What analytical techniques are used to characterize platinum dioxide?
A14: Various techniques are employed to characterize platinum dioxide, including X-ray diffraction (XRD), atomic force microscopy (AFM), X-ray photoelectron spectroscopy (XPS), surface photovoltage spectroscopy (SPS), electric field-induced surface photovoltage spectroscopy (FISPS), UV-Vis diffuse reflectance spectrophotometry (UV-Vis), transmission electron microscopy (TEM), and selected area electron diffraction (SAED). [, , , , , ]
Q15: How can the formation of platinum nanoparticles from platinum dioxide be monitored?
A15: Dynamic light scattering (DLS) is a valuable technique for monitoring the formation of platinum nanoparticles in solution. This method allows for real-time tracking of particle size distribution during the process. []
Q16: Can deuterium nuclear magnetic resonance spectroscopy be used to analyze compounds synthesized using platinum dioxide?
A16: Yes, deuterium nuclear magnetic resonance (2H NMR) spectroscopy is a powerful tool for determining the regiospecificity and extent of deuterium labeling in compounds. This technique has been used to analyze the distribution of deuterium in various nitrogen heterocyclic compounds after being labeled using pre-reduced platinum dioxide and heavy water. [, ]
Q17: What is the environmental impact of using platinum dioxide in industrial processes?
A17: Platinum is a precious metal with limited availability. While platinum dioxide itself might not pose significant environmental risks, the mining and refining of platinum can have adverse ecological consequences. Therefore, efficient recycling and waste management strategies are crucial for minimizing the environmental impact associated with using platinum-based catalysts like platinum dioxide. []
Avertissement et informations sur les produits de recherche in vitro
Veuillez noter que tous les articles et informations sur les produits présentés sur BenchChem sont destinés uniquement à des fins informatives. Les produits disponibles à l'achat sur BenchChem sont spécifiquement conçus pour des études in vitro, qui sont réalisées en dehors des organismes vivants. Les études in vitro, dérivées du terme latin "in verre", impliquent des expériences réalisées dans des environnements de laboratoire contrôlés à l'aide de cellules ou de tissus. Il est important de noter que ces produits ne sont pas classés comme médicaments et n'ont pas reçu l'approbation de la FDA pour la prévention, le traitement ou la guérison de toute condition médicale, affection ou maladie. Nous devons souligner que toute forme d'introduction corporelle de ces produits chez les humains ou les animaux est strictement interdite par la loi. Il est essentiel de respecter ces directives pour assurer la conformité aux normes légales et éthiques en matière de recherche et d'expérimentation.