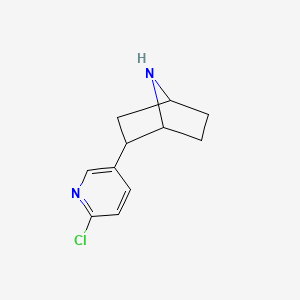
Epibatidine
Vue d'ensemble
Description
L’épibatidine est un alcaloïde puissant qui a été isolé pour la première fois de la peau de la grenouille équatorienne, Epipedobates anthonyi, en 1974 par John W. Daly . Ce composé est connu pour ses puissantes propriétés analgésiques, qui sont significativement plus puissantes que la morphine. La structure de l’épibatidine a été entièrement élucidée en 1992, révélant une fraction de pyridine chlorée connectée à une structure 7-azabicyclo[2.2.1]heptane .
Méthodes De Préparation
Voies de Synthèse et Conditions de Réaction : L’épibatidine peut être synthétisée par différentes méthodes. Une approche notable implique la synthèse formelle chimio-enzymatique, qui comprend la cis-dihydroxylation enzymatique du bromobenzène à l’aide de Pseudomonas putida UV4, suivie de plusieurs étapes pour obtenir le produit final . Une autre méthode implique la désymétrisation asymétrique, la protonation asymétrique et les réactions de Diels–Alder avec des allènes chiraux .
Méthodes de Production Industrielle : La production industrielle de l’épibatidine est difficile en raison de sa structure complexe et de la nécessité d’une haute stéréosélectivité. des méthodes à l’échelle préparative ont été développées, produisant à la fois des isomères endo et exo avec une efficacité raisonnable .
Analyse Des Réactions Chimiques
Types de Réactions : L’épibatidine subit diverses réactions chimiques, notamment l’oxydation, la réduction et la substitution. Ces réactions sont cruciales pour modifier sa structure et améliorer ses propriétés pharmacologiques.
Réactifs et Conditions Communs : Les réactifs courants utilisés dans la synthèse et la modification de l’épibatidine comprennent les catalyseurs au palladium, les auxiliaires chiraux et divers solvants organiques. Les conditions de réaction impliquent souvent des températures et des pressions contrôlées pour garantir des rendements élevés et une sélectivité .
Produits Principaux : Les produits principaux formés à partir de ces réactions sont généralement des analogues de l’épibatidine avec des profils pharmacologiques modifiés. Ces analogues sont étudiés pour leurs applications thérapeutiques potentielles et leur toxicité réduite .
4. Applications de la Recherche Scientifique
L’épibatidine a été largement étudiée pour ses applications dans divers domaines :
Chimie : En chimie, l’épibatidine sert de composé modèle pour étudier la synthèse d’alcaloïdes complexes et le développement de nouvelles méthodologies de synthèse .
Biologie : En recherche biologique, l’épibatidine est utilisée pour étudier la fonction des récepteurs nicotiniques de l’acétylcholine (nAChRs), qui jouent un rôle crucial dans la neurotransmission .
Médecine : Les puissantes propriétés analgésiques de l’épibatidine en ont fait un sujet d’intérêt dans la recherche sur la gestion de la douleur. Cependant, sa forte toxicité a limité son utilisation thérapeutique. Les chercheurs développent des analogues synthétiques avec des profils de sécurité améliorés .
Industrie : Dans l’industrie pharmaceutique, l’épibatidine et ses analogues sont explorés pour leur potentiel en tant que nouveaux médicaments analgésiques. La structure unique du composé et son mécanisme d’action fournissent des informations précieuses pour le développement de médicaments .
Applications De Recherche Scientifique
Pharmacological Properties
Epibatidine exhibits high affinity for nicotinic acetylcholine receptors, particularly the α4β2 and α3β4 subtypes, which are prevalent in the central nervous system. Its binding affinity is notably higher than that of traditional analgesics, making it a candidate for non-opioid pain relief strategies.
Key Characteristics:
- High Affinity : Ki = 0.12 nM for nicotinic receptors; low affinity for opioid receptors (Ki > 3.0 µM) .
- Analgesic Properties : Demonstrated short-lived analgesic effects in animal models without the side effects commonly associated with opioids .
Applications in Pain Management
This compound has been primarily studied for its analgesic potential. It acts as a non-opioid analgesic, providing pain relief through mechanisms distinct from traditional opioids.
Case Studies and Findings:
- In Vivo Studies :
- Mechanism of Action :
Neuroprotective Effects
Beyond pain management, this compound has demonstrated neuroprotective properties, suggesting potential applications in neurodegenerative diseases.
Research Insights:
- Neuroprotection :
Applications in Neurological Disorders
Recent studies have explored the use of this compound and its analogs in treating various neurological conditions.
Notable Developments:
- Cognitive Enhancement :
- Potential for Treating Drug Dependence :
Comprehensive Data Table
The following table summarizes key studies on the applications of this compound:
Mécanisme D'action
L’épibatidine exerce ses effets en interagissant avec les récepteurs nicotiniques et muscariniques de l’acétylcholine. Elle agit comme un agoniste à ces récepteurs, conduisant à la transmission des sensations douloureuses et au contrôle des mouvements . La forte affinité du composé pour les nAChRs, en particulier le sous-type α4β2, est responsable de ses puissants effets analgésiques . son interaction avec ces récepteurs contribue également à sa toxicité, provoquant un engourdissement et une paralysie à des doses plus élevées .
Comparaison Avec Des Composés Similaires
L’épibatidine est unique en raison de sa forte puissance et de ses interactions spécifiques avec les récepteurs. Des composés similaires comprennent :
ABT-594 : Un analogue synthétique de l’épibatidine avec une sécurité et une sélectivité améliorées.
ABT-418 : Un autre analogue avec des applications thérapeutiques potentielles.
Nicotine : Partage un mécanisme d’action similaire mais est moins puissant.
Ces composés mettent en évidence le potentiel des analogues de l’épibatidine dans le développement de nouveaux médicaments analgésiques avec de meilleurs profils de sécurité.
Activité Biologique
Epibatidine is a potent alkaloid originally extracted from the skin of the Ecuadorian poison frog Epipedobates tricolor. This compound has garnered significant interest due to its high affinity for nicotinic acetylcholine receptors (nAChRs), particularly the α4β2 subtype, and its potential therapeutic applications, despite its toxicity. This article delves into the biological activity of this compound, exploring its mechanisms, pharmacokinetics, structure-activity relationships, and recent research findings.
This compound acts primarily as an agonist at nAChRs, which are integral to neurotransmission in the central nervous system (CNS). Its binding affinity is notably high for the α4β2 subtype, with an inhibitory constant () of approximately 40 pM, making it significantly more potent than nicotine . The compound also interacts with other nAChR subtypes, including α7 and α3β4, albeit with lower affinity .
Table 1: Binding Affinities of this compound at Various nAChR Subtypes
nAChR Subtype | (pM) |
---|---|
α4β2 | 40 |
α7 | 20,000 |
α3β4 | 1500 |
Pharmacological Effects
This compound exhibits a range of biological effects primarily mediated through its action on nAChRs. It has been shown to possess potent analgesic properties, being over 200 times more effective than morphine in eliciting pain relief responses in animal models . However, its therapeutic use is limited due to a narrow therapeutic window and significant side effects, including respiratory depression and cardiovascular complications .
Neuroprotective Effects
Recent studies indicate that this compound may have neuroprotective properties. For instance, it has been shown to upregulate fibroblast growth factor (FGF) mRNA in rat brains, suggesting a role in neuroprotection against oxidative stress . Additionally, it enhances the expression of heme oxygenase-1 (HO-1), a key enzyme involved in cytoprotection during oxidative stress conditions.
Structure-Activity Relationships (SAR)
The structure-activity relationship studies have focused on synthesizing analogues of this compound to improve its selectivity and reduce toxicity. Modifications have aimed at enhancing the therapeutic index while retaining or amplifying its analgesic properties. For example, certain synthetic derivatives have demonstrated improved selectivity for specific nAChR subtypes, potentially mitigating unwanted side effects associated with non-selective activation .
Table 2: Selected this compound Analogues and Their Properties
Analogue Name | Selectivity | Potency (relative to this compound) | Notes |
---|---|---|---|
Analogue A | α4β2 | 5x | Reduced side effects |
Analogue B | α3β4 | 10x | Enhanced analgesic effect |
Analogue C | Non-selective | 0.5x | Increased toxicity |
Disruption of Retinal Activity
In a study involving ferrets, this compound was shown to disrupt correlated retinal activity during specific developmental stages. This disruption highlights the compound's influence on cholinergic signaling within the retina and suggests potential implications for visual processing .
Toxicity and Safety Profile
Despite its promising biological activities, this compound's toxicity remains a critical concern. The compound's ability to activate multiple nAChR subtypes can lead to adverse effects across various physiological systems. Research indicates that modifications to its structure can yield derivatives with better safety profiles while maintaining efficacy .
Propriétés
IUPAC Name |
2-(6-chloropyridin-3-yl)-7-azabicyclo[2.2.1]heptane | |
---|---|---|
Source | PubChem | |
URL | https://pubchem.ncbi.nlm.nih.gov | |
Description | Data deposited in or computed by PubChem | |
InChI |
InChI=1S/C11H13ClN2/c12-11-4-1-7(6-13-11)9-5-8-2-3-10(9)14-8/h1,4,6,8-10,14H,2-3,5H2 | |
Source | PubChem | |
URL | https://pubchem.ncbi.nlm.nih.gov | |
Description | Data deposited in or computed by PubChem | |
InChI Key |
NLPRAJRHRHZCQQ-UHFFFAOYSA-N | |
Source | PubChem | |
URL | https://pubchem.ncbi.nlm.nih.gov | |
Description | Data deposited in or computed by PubChem | |
Canonical SMILES |
C1CC2C(CC1N2)C3=CN=C(C=C3)Cl | |
Source | PubChem | |
URL | https://pubchem.ncbi.nlm.nih.gov | |
Description | Data deposited in or computed by PubChem | |
Molecular Formula |
C11H13ClN2 | |
Source | PubChem | |
URL | https://pubchem.ncbi.nlm.nih.gov | |
Description | Data deposited in or computed by PubChem | |
DSSTOX Substance ID |
DTXSID30861409 | |
Record name | 2-(6-Chloropyridin-3-yl)-7-azabicyclo[2.2.1]heptane | |
Source | EPA DSSTox | |
URL | https://comptox.epa.gov/dashboard/DTXSID30861409 | |
Description | DSSTox provides a high quality public chemistry resource for supporting improved predictive toxicology. | |
Molecular Weight |
208.69 g/mol | |
Source | PubChem | |
URL | https://pubchem.ncbi.nlm.nih.gov | |
Description | Data deposited in or computed by PubChem | |
Synthesis routes and methods I
Procedure details
Synthesis routes and methods II
Procedure details
Retrosynthesis Analysis
AI-Powered Synthesis Planning: Our tool employs the Template_relevance Pistachio, Template_relevance Bkms_metabolic, Template_relevance Pistachio_ringbreaker, Template_relevance Reaxys, Template_relevance Reaxys_biocatalysis model, leveraging a vast database of chemical reactions to predict feasible synthetic routes.
One-Step Synthesis Focus: Specifically designed for one-step synthesis, it provides concise and direct routes for your target compounds, streamlining the synthesis process.
Accurate Predictions: Utilizing the extensive PISTACHIO, BKMS_METABOLIC, PISTACHIO_RINGBREAKER, REAXYS, REAXYS_BIOCATALYSIS database, our tool offers high-accuracy predictions, reflecting the latest in chemical research and data.
Strategy Settings
Precursor scoring | Relevance Heuristic |
---|---|
Min. plausibility | 0.01 |
Model | Template_relevance |
Template Set | Pistachio/Bkms_metabolic/Pistachio_ringbreaker/Reaxys/Reaxys_biocatalysis |
Top-N result to add to graph | 6 |
Feasible Synthetic Routes
Avertissement et informations sur les produits de recherche in vitro
Veuillez noter que tous les articles et informations sur les produits présentés sur BenchChem sont destinés uniquement à des fins informatives. Les produits disponibles à l'achat sur BenchChem sont spécifiquement conçus pour des études in vitro, qui sont réalisées en dehors des organismes vivants. Les études in vitro, dérivées du terme latin "in verre", impliquent des expériences réalisées dans des environnements de laboratoire contrôlés à l'aide de cellules ou de tissus. Il est important de noter que ces produits ne sont pas classés comme médicaments et n'ont pas reçu l'approbation de la FDA pour la prévention, le traitement ou la guérison de toute condition médicale, affection ou maladie. Nous devons souligner que toute forme d'introduction corporelle de ces produits chez les humains ou les animaux est strictement interdite par la loi. Il est essentiel de respecter ces directives pour assurer la conformité aux normes légales et éthiques en matière de recherche et d'expérimentation.