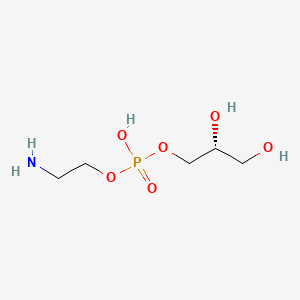
L-alpha-Glycerophosphorylethanolamine
Vue d'ensemble
Description
sn-glycero-3-phosphoethanolamine: is a glycerophospholipid characterized by an ethanolamine ester of glycerophosphoric acid. It is a cone-shaped lipid that can influence membrane curvature when incorporated into biological membranes . This compound is a main constituent of the phospholipid pool in many tissues, such as the liver and brain, and is the most abundant glycerophospholipid in most bacteria .
Applications De Recherche Scientifique
sn-glycero-3-phosphoethanolamine has a wide range of scientific research applications in chemistry, biology, medicine, and industry. In chemistry, it is used as a precursor for the synthesis of other glycerophospholipids . In biology, it plays a crucial role in membrane structure and function, influencing membrane curvature and fluidity . In medicine, it is used in the development of lipid-based drug delivery systems, such as lipid nanoparticles for mRNA delivery . In industry, it is used in the production of food additives and cognitive enhancers .
Mécanisme D'action
The mechanism of action of sn-glycero-3-phosphoethanolamine involves its incorporation into biological membranes, where it influences membrane curvature and fluidity . It is also a precursor for glycerophosphocholine via the phosphatidylethanolamine N-methyltransferase pathway, where it is methylated three times to yield glycerophosphocholine . Additionally, it is a precursor for anandamide, an endocannabinoid neurotransmitter .
Analyse Biochimique
Biochemical Properties
L-alpha-Glycerophosphorylethanolamine plays a significant role in various biochemical reactions. It interacts with several enzymes, proteins, and other biomolecules. For instance, it has been shown to interact with trimeric G alpha proteins and HRAS, where it hydrolyzes fatty acids from S-acylated cysteine residues . These interactions are essential for its function in cellular processes and its therapeutic effects.
Cellular Effects
This compound influences various types of cells and cellular processes. It has been observed to affect cell signaling pathways, gene expression, and cellular metabolism. For example, it can activate NF-κB and MAPK signaling pathways, leading to increased expression of pro-inflammatory effectors such as CXCL13 and TIMP-1 in human coronary artery endothelial cells . These effects highlight its potential impact on cellular function and its therapeutic relevance.
Molecular Mechanism
The molecular mechanism of this compound involves several binding interactions with biomolecules, enzyme inhibition or activation, and changes in gene expression. It can be metabolized into trimethylamine N-oxide, a pro-atherosclerotic agent, indicating its role in the progression of atherosclerosis . Additionally, it alters the gut microbiota composition, which further influences its molecular effects.
Temporal Effects in Laboratory Settings
In laboratory settings, the effects of this compound change over time. Its stability and degradation are crucial factors that influence its long-term effects on cellular function. Studies have shown that it can lead to changes in gut microbiota and inflammation over time, which are important considerations for its therapeutic use .
Dosage Effects in Animal Models
The effects of this compound vary with different dosages in animal models. At higher doses, it has been observed to increase the risk of stroke and promote atherosclerosis in hyperlipidemic mice . These findings suggest that careful dosage management is essential to avoid adverse effects while maximizing its therapeutic benefits.
Metabolic Pathways
This compound is involved in several metabolic pathways. It can be metabolized into trimethylamine N-oxide, which is associated with atherosclerosis . This compound also interacts with enzymes such as choline trimethylamine-lyase, which plays a role in its metabolic processing .
Transport and Distribution
Within cells and tissues, this compound is transported and distributed through various mechanisms. It interacts with transporters and binding proteins that facilitate its localization and accumulation in specific cellular compartments . These interactions are crucial for its function and therapeutic effects.
Subcellular Localization
This compound is localized in specific subcellular compartments, which affects its activity and function. It may undergo post-translational modifications that direct it to particular organelles, influencing its role in cellular processes . Understanding its subcellular localization is essential for elucidating its mechanism of action.
Méthodes De Préparation
Synthetic Routes and Reaction Conditions: The synthesis of sn-glycero-3-phosphoethanolamine in mammals can follow different pathways. Minor pathways include the acylation of monoacyl-glycerophosphoethanolamine by lyso-phosphatidylethanolamine-acyltransferase and a base exchange reaction between glycerophosphoserine and ethanolamine .
Industrial Production Methods: Industrial production methods for sn-glycero-3-phosphoethanolamine involve chemical and enzymatic approaches. Chemical methods include the use of sodium methoxide in chloroform and methanol, followed by purification through flash column chromatography . Enzymatic methods involve the use of specific enzymes to catalyze the formation of sn-glycero-3-phosphoethanolamine from its precursors .
Analyse Des Réactions Chimiques
Types of Reactions: sn-glycero-3-phosphoethanolamine undergoes various chemical reactions, including oxidation, reduction, and substitution reactions. These reactions can modify the fatty acid chains attached to the glycerol backbone or the ethanolamine head group .
Common Reagents and Conditions: Common reagents used in these reactions include sodium methoxide, chloroform, and methanol for chemical synthesis . Enzymatic reactions may involve specific enzymes such as lyso-phosphatidylethanolamine-acyltransferase .
Major Products: The major products formed from these reactions include modified glycerophosphoethanolamines with different fatty acid chains or head groups. These modifications can alter the physical and chemical properties of the compound, making it suitable for various applications .
Comparaison Avec Des Composés Similaires
Similar Compounds: Similar compounds to sn-glycero-3-phosphoethanolamine include phosphatidylcholine, phosphatidylinositol, and phosphatidylserine . These compounds are also glycerophospholipids with different head groups attached to the glycerophosphoric acid backbone.
Uniqueness: sn-glycero-3-phosphoethanolamine is unique due to its ethanolamine head group, which gives it distinct physical and chemical properties compared to other glycerophospholipids . Its ability to influence membrane curvature and fluidity makes it particularly important in biological membranes .
Propriétés
IUPAC Name |
2-aminoethyl [(2R)-2,3-dihydroxypropyl] hydrogen phosphate | |
---|---|---|
Source | PubChem | |
URL | https://pubchem.ncbi.nlm.nih.gov | |
Description | Data deposited in or computed by PubChem | |
InChI |
InChI=1S/C5H14NO6P/c6-1-2-11-13(9,10)12-4-5(8)3-7/h5,7-8H,1-4,6H2,(H,9,10)/t5-/m1/s1 | |
Source | PubChem | |
URL | https://pubchem.ncbi.nlm.nih.gov | |
Description | Data deposited in or computed by PubChem | |
InChI Key |
JZNWSCPGTDBMEW-RXMQYKEDSA-N | |
Source | PubChem | |
URL | https://pubchem.ncbi.nlm.nih.gov | |
Description | Data deposited in or computed by PubChem | |
Canonical SMILES |
C(COP(=O)(O)OCC(CO)O)N | |
Source | PubChem | |
URL | https://pubchem.ncbi.nlm.nih.gov | |
Description | Data deposited in or computed by PubChem | |
Isomeric SMILES |
C(COP(=O)(O)OC[C@@H](CO)O)N | |
Source | PubChem | |
URL | https://pubchem.ncbi.nlm.nih.gov | |
Description | Data deposited in or computed by PubChem | |
Molecular Formula |
C5H14NO6P | |
Source | PubChem | |
URL | https://pubchem.ncbi.nlm.nih.gov | |
Description | Data deposited in or computed by PubChem | |
Molecular Weight |
215.14 g/mol | |
Source | PubChem | |
URL | https://pubchem.ncbi.nlm.nih.gov | |
Description | Data deposited in or computed by PubChem | |
CAS RN |
33049-08-0 | |
Record name | alpha-Glycerophosphorylethanolamine, (R)- | |
Source | ChemIDplus | |
URL | https://pubchem.ncbi.nlm.nih.gov/substance/?source=chemidplus&sourceid=0033049080 | |
Description | ChemIDplus is a free, web search system that provides access to the structure and nomenclature authority files used for the identification of chemical substances cited in National Library of Medicine (NLM) databases, including the TOXNET system. | |
Record name | L-alpha-Glycerophosphorylethanolamine | |
Source | DrugBank | |
URL | https://www.drugbank.ca/drugs/DB15872 | |
Description | The DrugBank database is a unique bioinformatics and cheminformatics resource that combines detailed drug (i.e. chemical, pharmacological and pharmaceutical) data with comprehensive drug target (i.e. sequence, structure, and pathway) information. | |
Explanation | Creative Common's Attribution-NonCommercial 4.0 International License (http://creativecommons.org/licenses/by-nc/4.0/legalcode) | |
Record name | .ALPHA.-GLYCEROPHOSPHORYLETHANOLAMINE, (R)- | |
Source | FDA Global Substance Registration System (GSRS) | |
URL | https://gsrs.ncats.nih.gov/ginas/app/beta/substances/O35QN01X8F | |
Description | The FDA Global Substance Registration System (GSRS) enables the efficient and accurate exchange of information on what substances are in regulated products. Instead of relying on names, which vary across regulatory domains, countries, and regions, the GSRS knowledge base makes it possible for substances to be defined by standardized, scientific descriptions. | |
Explanation | Unless otherwise noted, the contents of the FDA website (www.fda.gov), both text and graphics, are not copyrighted. They are in the public domain and may be republished, reprinted and otherwise used freely by anyone without the need to obtain permission from FDA. Credit to the U.S. Food and Drug Administration as the source is appreciated but not required. | |
Retrosynthesis Analysis
AI-Powered Synthesis Planning: Our tool employs the Template_relevance Pistachio, Template_relevance Bkms_metabolic, Template_relevance Pistachio_ringbreaker, Template_relevance Reaxys, Template_relevance Reaxys_biocatalysis model, leveraging a vast database of chemical reactions to predict feasible synthetic routes.
One-Step Synthesis Focus: Specifically designed for one-step synthesis, it provides concise and direct routes for your target compounds, streamlining the synthesis process.
Accurate Predictions: Utilizing the extensive PISTACHIO, BKMS_METABOLIC, PISTACHIO_RINGBREAKER, REAXYS, REAXYS_BIOCATALYSIS database, our tool offers high-accuracy predictions, reflecting the latest in chemical research and data.
Strategy Settings
Precursor scoring | Relevance Heuristic |
---|---|
Min. plausibility | 0.01 |
Model | Template_relevance |
Template Set | Pistachio/Bkms_metabolic/Pistachio_ringbreaker/Reaxys/Reaxys_biocatalysis |
Top-N result to add to graph | 6 |
Feasible Synthetic Routes
Avertissement et informations sur les produits de recherche in vitro
Veuillez noter que tous les articles et informations sur les produits présentés sur BenchChem sont destinés uniquement à des fins informatives. Les produits disponibles à l'achat sur BenchChem sont spécifiquement conçus pour des études in vitro, qui sont réalisées en dehors des organismes vivants. Les études in vitro, dérivées du terme latin "in verre", impliquent des expériences réalisées dans des environnements de laboratoire contrôlés à l'aide de cellules ou de tissus. Il est important de noter que ces produits ne sont pas classés comme médicaments et n'ont pas reçu l'approbation de la FDA pour la prévention, le traitement ou la guérison de toute condition médicale, affection ou maladie. Nous devons souligner que toute forme d'introduction corporelle de ces produits chez les humains ou les animaux est strictement interdite par la loi. Il est essentiel de respecter ces directives pour assurer la conformité aux normes légales et éthiques en matière de recherche et d'expérimentation.