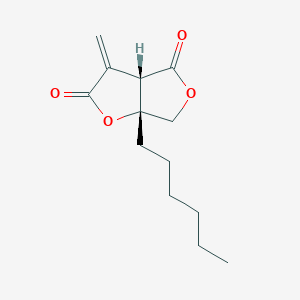
Sporothriolide
Vue d'ensemble
Description
Sporothriolide est un composé bioactif produit par certains champignons, en particulier ceux appartenant au genre Hypoxylon. Il appartient à la famille des furofurandiones et est connu pour ses puissantes propriétés antifongiques et cytotoxiques
Applications De Recherche Scientifique
Sporothriolide has a wide range of scientific research applications:
Mécanisme D'action
Target of Action
Sporothriolide is a secondary metabolite produced by certain ascomycetes, including Hypomontagnella monticulosa MUCL 54604, H. spongiphila CLL 205, and H. submonticulosa DAOMC 242471 .
Mode of Action
It is known that this compound is derived from non-enzymatic diels–alder cycloaddition of 1 and trienylfuranol a 7 during the fermentation and extraction process . This suggests that this compound may interact with its targets through a similar mechanism, potentially disrupting normal cellular processes in fungi.
Biochemical Pathways
The biosynthetic pathway of this compound involves the production of a dedicated decanoic acid by fungal FAS proteins, which is then reacted with oxaloacetate by a citrate synthase to give an octanyl citrate. This is then dehydrated to give octanyl aconitate and decarboxylated to the first observable intermediate, octanyl itaconic acid . This pathway originates in fatty acid biosynthesis .
Result of Action
This compound is known to have potent antifungal properties . It is also proposed to be a Diels Alder adduct of the furofurandione this compound 1, which possesses potent cytotoxicity against human cancer cell lines . This suggests that the molecular and cellular effects of this compound’s action may include disruption of fungal cellular processes and cytotoxic effects on cancer cells.
Action Environment
The action of this compound is likely influenced by environmental factors during the fermentation and extraction process . .
Analyse Biochimique
Biochemical Properties
Sporothriolide interacts with various biomolecules in biochemical reactions. The biosynthetic gene cluster of this compound was identified from three producing ascomycetes . Genes encoding a fatty acid synthase subunit and a citrate synthase were found to be crucial for the production of this compound .
Cellular Effects
This compound has significant effects on various types of cells and cellular processes. It is active against plant pathogenic fungi and certain algae, but not against bacteria like B. megaterium and E. coli . It inhibits mycelial growth of the plant pathogenic fungus R. solani .
Molecular Mechanism
The molecular mechanism of this compound involves its interaction with fatty acid synthase and citrate synthase . The sporochartines are derived from non-enzymatic Diels–Alder cycloaddition of this compound and trienylfuranol A 7 during the fermentation and extraction process .
Temporal Effects in Laboratory Settings
The effects of this compound change over time in laboratory settings. The production of this compound and sporochartine was lost when genes encoding a fatty acid synthase subunit and a citrate synthase were simultaneously knocked out .
Metabolic Pathways
This compound is involved in a new fungal biosynthetic pathway originating in fatty acid biosynthesis . The pathway involves the interaction of this compound with a fatty acid synthase subunit and a citrate synthase .
Méthodes De Préparation
Voies de synthèse et conditions de réaction
La synthèse totale de la sporothriolide a été réalisée en sept étapes à partir d'un acide carboxylique β,γ-insaturé disponible dans le commerce . Les étapes clés de cette synthèse comprennent :
Addition de Michael diastéréosélective : Un dérivé chiral d'oxazolidinone est ajouté à une nitro-oléfine.
Utilisation du cycle aromatique : Le cycle aromatique est utilisé comme fonctionnalité d'acide carboxylique masqué.
Élimination favorisée par la base : L'acide nitreux est éliminé pour installer l'unité lactone α-méthylène de la this compound dans l'étape finale.
Méthodes de production industrielle
La production industrielle de la this compound implique la fermentation de champignons tels que Hypoxylon monticulosum. Le groupe de gènes biosynthétiques responsable de la production de this compound a été identifié, et l'expression hétérologue dans Aspergillus oryzae a été utilisée pour produire des intermédiaires et délimiter la voie biosynthétique .
Analyse Des Réactions Chimiques
Types de réactions
La sporothriolide subit plusieurs types de réactions chimiques, notamment :
Cycloaddition de Diels-Alder : Cycloaddition de Diels-Alder non enzymatique avec le triénylfuranol A pendant la fermentation et l'extraction.
Oxydation et réduction : Implique un traitement oxydatif des intermédiaires précoces de citrate alkylique.
Réactifs et conditions courants
Réactifs : Dérivés chiraux d'oxazolidinone, nitro-oléfines, cycles aromatiques.
Conditions : Élimination favorisée par la base, addition de Michael diastéréosélective.
Principaux produits
Les principaux produits formés à partir de ces réactions comprennent la this compound elle-même et ses dérivés tels que l'acide sporothrique, l'acide isosporothrique et l'acide dihydroisosporothrique .
Applications de recherche scientifique
La this compound a un large éventail d'applications de recherche scientifique :
Chimie : Utilisée comme composé modèle pour étudier les réactions de Diels-Alder et les voies biosynthétiques.
Agriculture : Efficace contre les agents pathogènes des plantes, ce qui suggère son utilisation comme biopesticide.
Mécanisme d'action
La this compound exerce ses effets par plusieurs mécanismes :
Comparaison Avec Des Composés Similaires
La sporothriolide est unique par rapport aux autres composés similaires en raison de sa voie biosynthétique spécifique et de ses activités biologiques puissantes. Parmi les composés similaires, on peut citer :
Acide piliformique : Un autre métabolite fongique présentant des propriétés antifongiques.
Tyromycine : Connu pour son activité cytotoxique.
Acide byssochlamique : Un maléimide cyclique présentant des activités biologiques.
Ces composés présentent certaines similitudes structurelles avec la this compound, mais diffèrent par leurs origines biosynthétiques et leurs activités biologiques spécifiques.
Propriétés
Numéro CAS |
154799-92-5 |
---|---|
Formule moléculaire |
C13H18O4 |
Poids moléculaire |
238.28 g/mol |
Nom IUPAC |
(3aS,6R,6aR)-6-hexyl-3-methylidene-6,6a-dihydro-3aH-furo[3,4-b]furan-2,4-dione |
InChI |
InChI=1S/C13H18O4/c1-3-4-5-6-7-9-11-10(13(15)16-9)8(2)12(14)17-11/h9-11H,2-7H2,1H3/t9-,10+,11+/m1/s1 |
Clé InChI |
AENZSPQGLJVLND-VWYCJHECSA-N |
SMILES |
CCCCCCC12COC(=O)C1C(=C)C(=O)O2 |
SMILES isomérique |
CCCCCC[C@@H]1[C@H]2[C@H](C(=C)C(=O)O2)C(=O)O1 |
SMILES canonique |
CCCCCCC1C2C(C(=C)C(=O)O2)C(=O)O1 |
154799-92-5 | |
Synonymes |
6-n-hexyl-3,3a,6,6a-tetrahydro-3-methylenefuro(3,4-b)furan-2,4-dione sporothriolide |
Origine du produit |
United States |
Retrosynthesis Analysis
AI-Powered Synthesis Planning: Our tool employs the Template_relevance Pistachio, Template_relevance Bkms_metabolic, Template_relevance Pistachio_ringbreaker, Template_relevance Reaxys, Template_relevance Reaxys_biocatalysis model, leveraging a vast database of chemical reactions to predict feasible synthetic routes.
One-Step Synthesis Focus: Specifically designed for one-step synthesis, it provides concise and direct routes for your target compounds, streamlining the synthesis process.
Accurate Predictions: Utilizing the extensive PISTACHIO, BKMS_METABOLIC, PISTACHIO_RINGBREAKER, REAXYS, REAXYS_BIOCATALYSIS database, our tool offers high-accuracy predictions, reflecting the latest in chemical research and data.
Strategy Settings
Precursor scoring | Relevance Heuristic |
---|---|
Min. plausibility | 0.01 |
Model | Template_relevance |
Template Set | Pistachio/Bkms_metabolic/Pistachio_ringbreaker/Reaxys/Reaxys_biocatalysis |
Top-N result to add to graph | 6 |
Feasible Synthetic Routes
Q1: What is Sporothriolide and where is it found?
A1: this compound is a natural antifungal metabolite primarily produced by fungi of the genus Hypomontagnella, formerly classified as Hypoxylon. [, , ] It was initially isolated from Sporothrix sp., Discosia sp., and Pezicula livida. [, , ]
Q2: Which fungi are known to produce this compound?
A2: this compound production appears to be a characteristic feature of Hypomontagnella monticulosa and has not been observed in other Hypoxylon species. [, ] Other this compound-producing fungi include Hypomontagnella spongiphila and Hypomontagnella submonticulosa. []
Q3: What is the molecular formula and weight of this compound?
A3: Unfortunately, the provided research excerpts do not explicitly state the molecular formula and weight of this compound. Further investigation into the chemical structure and properties of this compound from the original research articles would be necessary to provide this information.
Q4: What is the chemical structure of this compound?
A4: this compound belongs to the furofurandione class of compounds. [] Its core structure consists of a bicyclic lactone system. Several research papers describe the total synthesis of this compound, highlighting key structural features and methods for their construction. [, , , , , , , ]
Q5: How is this compound biosynthesized?
A5: Research suggests that this compound biosynthesis originates from fatty acid biosynthesis. [] A gene cluster responsible for its production has been identified in Hypomontagnella species. Knock-out experiments targeting genes within this cluster, specifically those encoding a fatty acid synthase subunit and a citrate synthase, resulted in the loss of this compound production. []
Q6: Are there any known derivatives of this compound?
A6: Yes, several this compound derivatives have been identified. These include:
- Sporothric acid []
- Isosporothric acid []
- Dihydroisosporothric acid []
- Sporothioethers A and B []
- A deoxy analogue of sporothric acid []
- A novel compound combining this compound and trienylfuranol A moieties []
Q7: What are Sporochartines, and how are they related to this compound?
A8: Sporochartines (A-D) are compounds formed through a non-enzymatic Diels-Alder cycloaddition reaction between this compound and trienylfuranol A. [, ] This reaction is believed to occur during the fermentation and extraction process of this compound-producing fungi. []
Q8: Have any synthetic routes for this compound been developed?
A8: Yes, several total syntheses of this compound have been reported, employing various strategies such as:
Avertissement et informations sur les produits de recherche in vitro
Veuillez noter que tous les articles et informations sur les produits présentés sur BenchChem sont destinés uniquement à des fins informatives. Les produits disponibles à l'achat sur BenchChem sont spécifiquement conçus pour des études in vitro, qui sont réalisées en dehors des organismes vivants. Les études in vitro, dérivées du terme latin "in verre", impliquent des expériences réalisées dans des environnements de laboratoire contrôlés à l'aide de cellules ou de tissus. Il est important de noter que ces produits ne sont pas classés comme médicaments et n'ont pas reçu l'approbation de la FDA pour la prévention, le traitement ou la guérison de toute condition médicale, affection ou maladie. Nous devons souligner que toute forme d'introduction corporelle de ces produits chez les humains ou les animaux est strictement interdite par la loi. Il est essentiel de respecter ces directives pour assurer la conformité aux normes légales et éthiques en matière de recherche et d'expérimentation.