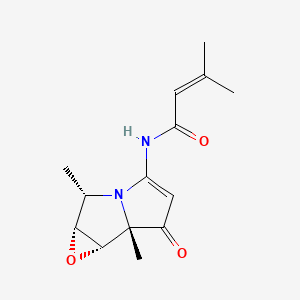
Bohemamine
Vue d'ensemble
Description
Bohemamine is a naturally occurring alkaloid compound that was first isolated from the leaves of the Bohemian plant (Aconitum bohemicum), a member of the Ranunculaceae family. It has been used in traditional medicine for centuries, but its chemical structure and biological activity were only recently discovered. This compound is a unique alkaloid and has been found to possess a variety of pharmacological activities, including anti-inflammatory, anti-microbial, anti-oxidant, anti-cancer, and anti-diabetic properties. This review will discuss the synthesis methods, scientific research applications, mechanism of action, biochemical and physiological effects, advantages and limitations for lab experiments, and future directions for this compound research.
Applications De Recherche Scientifique
Découverte de médicaments d'origine marine
Des dérivés de la bohemamine ont été isolés à partir de Streptomyces spinoverrucosus d'origine marine {svg_1}. Ces composés, appelés Spithionéines A et B, sont des produits naturels rares qui intègrent l'acide aminé ergothionéine {svg_2}. Cette découverte a suscité un intérêt pour le rôle potentiel de ces molécules en tant que thérapeutiques {svg_3}.
Activité antioxydante
Les dérivés de la this compound ont montré une activité antioxydante significative {svg_4}. Plus précisément, les composés 13, 15, 19 et 21, qui ont été synthétisés par une stratégie de chimère de produit naturel, ont présenté cette propriété {svg_5}. Cela suggère des applications potentielles dans la lutte contre les maladies liées au stress oxydatif {svg_6}.
Recherche sur la biosynthèse
La this compound est impliquée dans la biosynthèse des alcaloïdes pyrrolizidiniques {svg_7}. L'étude de ses voies biosynthétiques pourrait fournir des informations sur la production de ces alcaloïdes, qui possèdent diverses activités biologiques {svg_8}.
Investigation de la structure chimique
La structure chimique de la this compound J, un dérivé de la this compound, a été élucidée {svg_9}. Elle possède un nouveau squelette chimérique dérivé de la this compound A et du phénylacétaldéhyde {svg_10}. Cette investigation structurale peut contribuer au développement de nouvelles stratégies de synthèse et à la découverte de nouveaux composés {svg_11}.
Stratégie de chimère de produit naturel
La this compound a été utilisée dans la synthèse de dérivés chimériques par une stratégie de chimère de produit naturel {svg_12}. Cette approche peut conduire à la création de nouveaux composés présentant des activités biologiques potentiellement utiles {svg_13}.
Production d'actinomycine D
La this compound est impliquée dans la production d'actinomycine D, un agent chimiothérapeutique {svg_14}. Comprendre son rôle dans ce processus pourrait améliorer la production de ce médicament important {svg_15}.
Mécanisme D'action
Target of Action
Bohemamines (BHMs) are bacterial alkaloids containing a pyrrolizidine core The primary targets of Bohemamine are not explicitly mentioned in the available literature
Mode of Action
The biosynthesis of Bohemamines involves a coordinated action of nonribosomal peptide synthetase BhmJ, Baeyer-Villiger monooxygenase BhmK, and methyltransferase BhmG . BhmJ is responsible for incorporating a nonproteinogenic amino acid (2S,5S)-5-methyl-proline (5-Mepro). The tandem action of BhmK and BhmG is responsible for the formation of the pyrrolizidine core and C-7 methylation .
Biochemical Pathways
The biosynthesis of Bohemamines involves nonribosomal peptide synthetases (NRPSs) for the formation of indolizidine intermediates, followed by consecutive reactions to generate pyrrolizidine scaffolds by Baeyer-Villiger monooxygenases (BVMOs)
Result of Action
Bohemamines have been found to have cytotoxicity against cancer cell lines . Dibohemamines B and C, two new this compound analogues, were found to have nanomolar cytotoxicity against the non-small cell-lung cancer cell line A549 .
Action Environment
The production of Bohemamines is activated using a ribosome engineering approach in Streptomyces sp. CB02009 . The highly unstable nature of some intermediates in the biosynthesis of Bohemamines suggests that the coordinated action of BhmJ, BhmK, and BhmG might be one of Nature’s strategies to avoid the accumulation of shunt metabolites . This indicates that the action, efficacy, and stability of this compound could be influenced by environmental factors such as the conditions of the bacterial culture.
Analyse Biochimique
Biochemical Properties
Bohemamine plays a crucial role in biochemical reactions, particularly in its interactions with enzymes and proteins. It has been observed to interact with various biomolecules, including enzymes involved in DNA replication and repair. This compound binds to DNA, inhibiting the activity of topoisomerase II, an enzyme essential for DNA replication . This interaction leads to the stabilization of the DNA-topoisomerase II complex, preventing the re-ligation of DNA strands and ultimately causing cell death. Additionally, this compound has been shown to interact with proteins involved in cell signaling pathways, further influencing cellular functions.
Cellular Effects
This compound exerts significant effects on various types of cells and cellular processes. In cancer cells, this compound induces apoptosis by disrupting the mitochondrial membrane potential and activating caspase-dependent pathways . It also affects cell signaling pathways, such as the MAPK/ERK pathway, leading to altered gene expression and inhibition of cell proliferation. In normal cells, this compound can influence cellular metabolism by modulating the activity of metabolic enzymes, thereby affecting energy production and utilization.
Molecular Mechanism
The molecular mechanism of this compound involves its binding interactions with biomolecules and enzyme inhibition. This compound binds to DNA, forming a stable complex with topoisomerase II, which inhibits the enzyme’s activity and prevents DNA replication . This inhibition leads to the accumulation of DNA breaks and triggers cell death. Additionally, this compound can modulate gene expression by interacting with transcription factors and altering their binding to DNA. This results in changes in the expression of genes involved in cell cycle regulation, apoptosis, and stress responses.
Temporal Effects in Laboratory Settings
In laboratory settings, the effects of this compound have been observed to change over time. This compound is relatively stable under standard laboratory conditions, but its degradation can occur over extended periods . Studies have shown that this compound retains its biological activity for several days when stored at low temperatures. Prolonged exposure to higher temperatures can lead to its degradation and reduced efficacy. Long-term effects of this compound on cellular function have been observed in both in vitro and in vivo studies, with sustained exposure leading to cumulative cellular damage and altered metabolic processes.
Dosage Effects in Animal Models
The effects of this compound vary with different dosages in animal models. At low doses, this compound exhibits antitumor activity by inhibiting tumor growth and inducing apoptosis in cancer cells . At higher doses, this compound can cause toxic effects, including hepatotoxicity and nephrotoxicity. Threshold effects have been observed, where a certain dosage is required to achieve therapeutic benefits without causing significant adverse effects. The therapeutic window of this compound is narrow, necessitating careful dosage optimization in preclinical studies.
Metabolic Pathways
This compound is involved in several metabolic pathways, interacting with enzymes and cofactors essential for its metabolism. It undergoes biotransformation in the liver, where it is metabolized by cytochrome P450 enzymes . The metabolites of this compound can further interact with cellular components, influencing metabolic flux and altering metabolite levels. These interactions can affect various physiological processes, including detoxification, energy production, and cellular signaling.
Transport and Distribution
This compound is transported and distributed within cells and tissues through specific transporters and binding proteins. It can cross cell membranes via passive diffusion and active transport mechanisms . Once inside the cell, this compound can bind to intracellular proteins, influencing its localization and accumulation. The distribution of this compound within tissues is influenced by factors such as blood flow, tissue permeability, and binding affinity to cellular components.
Subcellular Localization
The subcellular localization of this compound is critical for its activity and function. This compound is primarily localized in the nucleus, where it interacts with DNA and nuclear proteins . It can also be found in the cytoplasm, where it may interact with cytoplasmic proteins and organelles. The targeting of this compound to specific subcellular compartments is facilitated by post-translational modifications and targeting signals that direct it to the nucleus or other organelles. These localizations are essential for this compound’s role in modulating cellular processes and exerting its biological effects.
Propriétés
IUPAC Name |
N-[(1S,2S,4R,5S)-1,5-dimethyl-9-oxo-3-oxa-6-azatricyclo[4.3.0.02,4]non-7-en-7-yl]-3-methylbut-2-enamide | |
---|---|---|
Source | PubChem | |
URL | https://pubchem.ncbi.nlm.nih.gov | |
Description | Data deposited in or computed by PubChem | |
InChI |
InChI=1S/C14H18N2O3/c1-7(2)5-11(18)15-10-6-9(17)14(4)13-12(19-13)8(3)16(10)14/h5-6,8,12-13H,1-4H3,(H,15,18)/t8-,12+,13+,14+/m0/s1 | |
Source | PubChem | |
URL | https://pubchem.ncbi.nlm.nih.gov | |
Description | Data deposited in or computed by PubChem | |
InChI Key |
UARXZADXFDTXOF-OKVQYTGBSA-N | |
Source | PubChem | |
URL | https://pubchem.ncbi.nlm.nih.gov | |
Description | Data deposited in or computed by PubChem | |
Canonical SMILES |
CC1C2C(O2)C3(N1C(=CC3=O)NC(=O)C=C(C)C)C | |
Source | PubChem | |
URL | https://pubchem.ncbi.nlm.nih.gov | |
Description | Data deposited in or computed by PubChem | |
Isomeric SMILES |
C[C@H]1[C@@H]2[C@@H](O2)[C@@]3(N1C(=CC3=O)NC(=O)C=C(C)C)C | |
Source | PubChem | |
URL | https://pubchem.ncbi.nlm.nih.gov | |
Description | Data deposited in or computed by PubChem | |
Molecular Formula |
C14H18N2O3 | |
Source | PubChem | |
URL | https://pubchem.ncbi.nlm.nih.gov | |
Description | Data deposited in or computed by PubChem | |
DSSTOX Substance ID |
DTXSID80993704 | |
Record name | Bohemamine | |
Source | EPA DSSTox | |
URL | https://comptox.epa.gov/dashboard/DTXSID80993704 | |
Description | DSSTox provides a high quality public chemistry resource for supporting improved predictive toxicology. | |
Molecular Weight |
262.30 g/mol | |
Source | PubChem | |
URL | https://pubchem.ncbi.nlm.nih.gov | |
Description | Data deposited in or computed by PubChem | |
CAS RN |
72926-12-6 | |
Record name | Bohemamine | |
Source | ChemIDplus | |
URL | https://pubchem.ncbi.nlm.nih.gov/substance/?source=chemidplus&sourceid=0072926126 | |
Description | ChemIDplus is a free, web search system that provides access to the structure and nomenclature authority files used for the identification of chemical substances cited in National Library of Medicine (NLM) databases, including the TOXNET system. | |
Record name | Bohemamine | |
Source | EPA DSSTox | |
URL | https://comptox.epa.gov/dashboard/DTXSID80993704 | |
Description | DSSTox provides a high quality public chemistry resource for supporting improved predictive toxicology. | |
Retrosynthesis Analysis
AI-Powered Synthesis Planning: Our tool employs the Template_relevance Pistachio, Template_relevance Bkms_metabolic, Template_relevance Pistachio_ringbreaker, Template_relevance Reaxys, Template_relevance Reaxys_biocatalysis model, leveraging a vast database of chemical reactions to predict feasible synthetic routes.
One-Step Synthesis Focus: Specifically designed for one-step synthesis, it provides concise and direct routes for your target compounds, streamlining the synthesis process.
Accurate Predictions: Utilizing the extensive PISTACHIO, BKMS_METABOLIC, PISTACHIO_RINGBREAKER, REAXYS, REAXYS_BIOCATALYSIS database, our tool offers high-accuracy predictions, reflecting the latest in chemical research and data.
Strategy Settings
Precursor scoring | Relevance Heuristic |
---|---|
Min. plausibility | 0.01 |
Model | Template_relevance |
Template Set | Pistachio/Bkms_metabolic/Pistachio_ringbreaker/Reaxys/Reaxys_biocatalysis |
Top-N result to add to graph | 6 |
Feasible Synthetic Routes
Q & A
Q1: What are bohemamines and where are they found?
A1: Bohemamines are a class of pyrrolizidine alkaloids primarily isolated from various Streptomyces species, many of which are marine-derived. [, , , , , , ] These bacteria are known for their ability to produce a diverse array of secondary metabolites.
Q2: What is unique about the chemical structure of bohemamines?
A2: Bohemamines possess a pyrrolizidine core with two unusual methyl groups at positions C-7 and C-9. [, ] The C-9 methyl group originates from a non-proteinogenic amino acid, (2S,5S)-5-methylproline, incorporated during biosynthesis. [] Interestingly, some bohemamine derivatives, like spithioneines A and B, incorporate the amino acid ergothioneine as a pyrrolizidine substituent, a rare occurrence in natural products. [, ]
Q3: How are bohemamines biosynthesized in Streptomyces?
A3: Studies on Streptomyces sp. CB02009 have revealed a fascinating biosynthetic pathway for bohemamines. The process involves a coordinated action of three key enzymes: a nonribosomal peptide synthetase (BhmJ), a Baeyer-Villiger monooxygenase (BhmK), and a methyltransferase (BhmG). [] BhmJ and BhmK are responsible for constructing the pyrrolizidine core. [] BhmG then specifically methylates the C-7 position. [] The C-9 methyl group is introduced through the incorporation of (2S,5S)-5-methylproline. [] This economical biosynthetic route highlights the intricate enzymatic interplay in this compound production.
Q4: Have any synthetic approaches been explored for this compound production?
A4: Yes, researchers have successfully synthesized dimeric this compound analogues (dibohemamines) through a non-enzymatic process. [] By reacting monomeric bohemamines with formaldehyde under mild conditions, they were able to mimic the dimerization observed in natural dibohemamines. [] This discovery not only confirmed the structures of naturally occurring dibohemamines but also paved the way for generating a library of this compound analogues for biological evaluation. []
Q5: What biological activities have been reported for bohemamines?
A5: Bohemamines have shown interesting biological activities, particularly cytotoxicity against cancer cell lines. For example, dithis compound F displayed potent cytotoxicity against A549 (lung cancer) and HepG2 (liver cancer) cell lines with IC50 values of 1.1 and 0.3 μM, respectively. [] Dibohemamines B and C also exhibited nanomolar cytotoxicity against the A549 cell line. [] Other this compound derivatives, like those isolated from Streptomyces sp. UMA-044, have been identified as inhibitors of cell adhesion mediated by LFA-1/ICAM-1 interactions. []
Q6: Are there any genomic insights into this compound production?
A6: The draft genome sequence of the marine-derived Streptomyces sp. TP-A0873, a known producer of this compound, has been determined. [] Analysis of the genome revealed the presence of at least 14 gene clusters potentially encoding polyketide synthases (PKS) and nonribosomal peptide synthetases (NRPS). [] These findings highlight the rich biosynthetic potential of this strain and provide a basis for further exploration of its secondary metabolite repertoire.
Q7: Has this compound been identified in organisms other than Streptomyces?
A7: While this compound itself has primarily been isolated from Streptomyces, a structurally similar compound, hennaminal, was discovered in the marine cyanobacterium Rivularia sp. [] Hennaminal shares the rare β,β-diamino unsaturated ketone functionality with this compound, suggesting a potential evolutionary link or convergent evolution of this chemical motif. []
Avertissement et informations sur les produits de recherche in vitro
Veuillez noter que tous les articles et informations sur les produits présentés sur BenchChem sont destinés uniquement à des fins informatives. Les produits disponibles à l'achat sur BenchChem sont spécifiquement conçus pour des études in vitro, qui sont réalisées en dehors des organismes vivants. Les études in vitro, dérivées du terme latin "in verre", impliquent des expériences réalisées dans des environnements de laboratoire contrôlés à l'aide de cellules ou de tissus. Il est important de noter que ces produits ne sont pas classés comme médicaments et n'ont pas reçu l'approbation de la FDA pour la prévention, le traitement ou la guérison de toute condition médicale, affection ou maladie. Nous devons souligner que toute forme d'introduction corporelle de ces produits chez les humains ou les animaux est strictement interdite par la loi. Il est essentiel de respecter ces directives pour assurer la conformité aux normes légales et éthiques en matière de recherche et d'expérimentation.