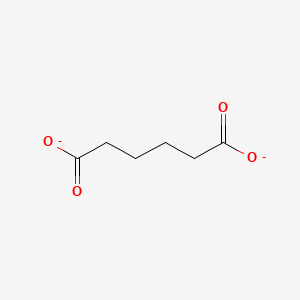
Adipate
Vue d'ensemble
Description
Adipate(2-) is a dicarboxylic acid dianion obtained by the deprotonation of both the carboxy groups of adipic acid. It has a role as a human xenobiotic metabolite. It is a conjugate base of an this compound(1-).
Activité Biologique
Adipates, particularly di(2-ethylhexyl) adipate (DEHA) and polyglycerol this compound (PGA), are esters derived from adipic acid that exhibit various biological activities. This article explores their biological effects, mechanisms of action, and applications in drug delivery systems, supported by relevant case studies and research findings.
Overview of this compound Compounds
Adipates are commonly used as plasticizers in various applications, including food packaging and medical devices. Among them, DEHA is notable for its widespread use in PVC products, while PGA is recognized for its biocompatibility and potential in biomedical applications.
Toxicological Studies
- Hepatic Effects : Research indicates that dietary administration of DEHA can lead to significant hepatic alterations. In a study involving Fischer 344 rats, feeding DEHA at concentrations of 1.0% to 2.0% resulted in increased liver weight and peroxisome proliferation. Specifically, the relative liver weight increased significantly at doses as low as 0.6% (1495 mg/kg bw per day) .
- Metabolism : DEHA is metabolized into adipic acid and mono(2-ethylhexyl) this compound (MEHA), with studies showing that approximately 20-30% of administered doses are excreted as adipic acid . This rapid metabolism suggests a low bioaccumulation potential.
- Endocrine Disruption : Some studies have raised concerns about the endocrine-disrupting potential of DEHA, although definitive conclusions remain elusive .
Table 1: Toxicological Data on DEHA
Endpoint | Findings |
---|---|
Hepatic Weight Increase | Significant at ≥0.6% diet |
Peroxisomal Proliferation | Dose-dependent increase observed |
Metabolite Excretion | 20-30% as adipic acid |
Biological Activity of Polyglycerol this compound (PGA)
PGA is synthesized through the polycondensation of glycerol and adipic acid, yielding a biodegradable polymer with unique properties suitable for drug delivery.
Drug Delivery Applications
- Nanoparticle Formation : PGA can self-assemble into nanoparticles (NPs) in aqueous environments, making it an excellent candidate for drug delivery systems . Studies have shown that PGA-grafted polycaprolactone (PCL) nanoparticles effectively encapsulate drugs like usnic acid, demonstrating prolonged release profiles .
- Biocompatibility : PGA exhibits high biocompatibility due to its biodegradable nature and functional hydroxyl groups that allow for further modifications . These characteristics enhance its suitability for medical applications.
- Release Mechanism : The release of drugs from PGA-based NPs follows the Korsmeyer–Peppas model, indicating a combination of diffusion and polymer degradation processes .
Table 2: Properties of Polyglycerol this compound Nanoparticles
Property | Value/Description |
---|---|
Biodegradability | Yes |
Self-assembly | Forms NPs in aqueous media |
Drug Release Duration | Prolonged release up to 172 hours |
Case Studies
- Case Study on DEHA : A study on the effects of DEHA on Wistar rats indicated increased hepatic fatty acid-binding protein levels after exposure to dietary concentrations . This finding highlights the compound's potential impact on lipid metabolism.
- Case Study on PGA : Research focusing on PGA-g-PCL nanoparticles demonstrated their effectiveness as carriers for usnic acid, achieving over 80% drug release while maintaining biocompatibility . The study emphasized the importance of side chain length in modulating drug release rates.
Propriétés
Numéro CAS |
764-65-8 |
---|---|
Formule moléculaire |
C6H8O4-2 |
Poids moléculaire |
144.12 g/mol |
Nom IUPAC |
hexanedioate |
InChI |
InChI=1S/C6H10O4/c7-5(8)3-1-2-4-6(9)10/h1-4H2,(H,7,8)(H,9,10)/p-2 |
Clé InChI |
WNLRTRBMVRJNCN-UHFFFAOYSA-L |
SMILES |
C(CCC(=O)[O-])CC(=O)[O-] |
SMILES canonique |
C(CCC(=O)[O-])CC(=O)[O-] |
Key on ui other cas no. |
764-65-8 |
Synonymes |
adipate adipic acid adipic acid, calcium salt adipic acid, Cu salt adipic acid, Cu(+2) salt adipic acid, Cu(+2) salt (1:1) adipic acid, diammonium salt adipic acid, disodium salt adipic acid, Mg salt (1:1) adipic acid, monoammonium salt adipic acid, nickel salt adipic acid, potassium salt adipic acid, sodium salt ammonium adipate diammonium adipate hexanedioic acid magnesium adipate sodium adipate |
Origine du produit |
United States |
Synthesis routes and methods
Procedure details
Retrosynthesis Analysis
AI-Powered Synthesis Planning: Our tool employs the Template_relevance Pistachio, Template_relevance Bkms_metabolic, Template_relevance Pistachio_ringbreaker, Template_relevance Reaxys, Template_relevance Reaxys_biocatalysis model, leveraging a vast database of chemical reactions to predict feasible synthetic routes.
One-Step Synthesis Focus: Specifically designed for one-step synthesis, it provides concise and direct routes for your target compounds, streamlining the synthesis process.
Accurate Predictions: Utilizing the extensive PISTACHIO, BKMS_METABOLIC, PISTACHIO_RINGBREAKER, REAXYS, REAXYS_BIOCATALYSIS database, our tool offers high-accuracy predictions, reflecting the latest in chemical research and data.
Strategy Settings
Precursor scoring | Relevance Heuristic |
---|---|
Min. plausibility | 0.01 |
Model | Template_relevance |
Template Set | Pistachio/Bkms_metabolic/Pistachio_ringbreaker/Reaxys/Reaxys_biocatalysis |
Top-N result to add to graph | 6 |
Feasible Synthetic Routes
Avertissement et informations sur les produits de recherche in vitro
Veuillez noter que tous les articles et informations sur les produits présentés sur BenchChem sont destinés uniquement à des fins informatives. Les produits disponibles à l'achat sur BenchChem sont spécifiquement conçus pour des études in vitro, qui sont réalisées en dehors des organismes vivants. Les études in vitro, dérivées du terme latin "in verre", impliquent des expériences réalisées dans des environnements de laboratoire contrôlés à l'aide de cellules ou de tissus. Il est important de noter que ces produits ne sont pas classés comme médicaments et n'ont pas reçu l'approbation de la FDA pour la prévention, le traitement ou la guérison de toute condition médicale, affection ou maladie. Nous devons souligner que toute forme d'introduction corporelle de ces produits chez les humains ou les animaux est strictement interdite par la loi. Il est essentiel de respecter ces directives pour assurer la conformité aux normes légales et éthiques en matière de recherche et d'expérimentation.