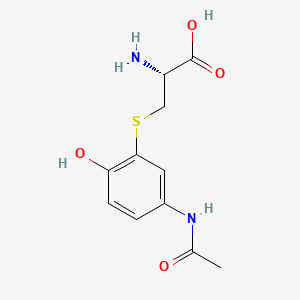
3-(Cystein-S-yl)paracétamol
Vue d'ensemble
Description
3-(Cystein-S-yl)acetaminophen, also known as paracetamol-cysteine or PCM-C, belongs to the class of organic compounds known as l-cysteine-s-conjugates. L-cysteine-S-conjugates are compounds containing L-cysteine where the thio-group is conjugated. 3-(Cystein-S-yl)acetaminophen is considered to be a practically insoluble (in water) and relatively neutral molecule.
Applications De Recherche Scientifique
Biomarqueur d'exposition au paracétamol
Les adduits acétaminophène-cystéine (APAP-CYS), tels que le 3-(Cystein-S-yl)paracétamol, sont un biomarqueur spécifique de l'exposition au paracétamol {svg_1}. Ils ont été décrits dans le cadre d'un surdosage aigu et une concentration >1,1 nmol/ml a été suggérée comme un marqueur de lésion hépatique due à un surdosage de paracétamol chez les patients présentant une ALT >1000 UI/L {svg_2}.
Indicateur d'hépatotoxicité
La présence de this compound peut indiquer une hépatotoxicité. En effet, il se forme lorsque le paracétamol est métabolisé en N-acétyl-p-benzoquinone imine et se lie aux groupes cystéine {svg_3}. Cet adduit peut être détecté avant l'apparition de la nécrose centrolobulaire {svg_4}.
Localisation dans les cellules hépatiques
Des études immunohistochimiques ont montré que le this compound peut être localisé dans les cellules hépatiques {svg_5}. Cette localisation se produit selon un schéma temporel progressif, central-périphérique au sein du lobule hépatique {svg_6}.
Indicateur de la liaison médicament-protéine
La présence de this compound peut indiquer une liaison médicament-protéine dans les hépatocytes à des doses subhépatotoxiques et avant l'épuisement du glutathion hépatique total {svg_7}.
Indicateur de la progression de la toxicité du paracétamol
Des zones lobulaires distinctes de localisation de l'adduit avec un épuisement ultérieur pendant la progression de la toxicité ont été observées {svg_8}. Cela peut fournir des informations précieuses sur la progression de la toxicité du paracétamol.
Présence dans les hépatocytes métaboliquement actifs et en division
Le this compound a été retrouvé dans les hépatocytes métaboliquement actifs et en division et dans les cellules de type macrophage dans le foie en régénération {svg_9}. Cela suggère un rôle dans les processus de régénération du foie.
Mécanisme D'action
- Central mechanisms include:
- Paracetamol’s downstream effects involve:
- At the molecular and cellular levels:
Target of Action
Mode of Action
Biochemical Pathways
Pharmacokinetics
Result of Action
Action Environment
Analyse Biochimique
Biochemical Properties
3-(Cystein-S-yl)paracetamol plays a significant role in biochemical reactions, particularly in the detoxification of paracetamol. This compound is formed when paracetamol is metabolized by cytochrome P450 enzymes, producing a reactive intermediate known as N-acetyl-p-benzoquinone imine (NAPQI). NAPQI is highly reactive and can cause cellular damage if not detoxified. The body neutralizes NAPQI by conjugating it with glutathione, forming 3-(Cystein-S-yl)paracetamol .
The enzymes involved in this process include cytochrome P450 2E1 (CYP2E1) and glutathione S-transferase. CYP2E1 is responsible for the initial oxidation of paracetamol to NAPQI, while glutathione S-transferase facilitates the conjugation of NAPQI with glutathione . This interaction is crucial for preventing the accumulation of toxic metabolites and protecting cells from oxidative damage.
Cellular Effects
3-(Cystein-S-yl)paracetamol has several effects on cellular processes. It is primarily involved in the detoxification of NAPQI, thereby preventing cellular damage. In cases of paracetamol overdose, the detoxification pathways can become overwhelmed, leading to the accumulation of NAPQI and subsequent cellular injury .
The compound influences cell function by modulating cell signaling pathways, gene expression, and cellular metabolism. For instance, the formation of 3-(Cystein-S-yl)paracetamol can trigger oxidative stress responses and activate signaling pathways involved in cell survival and apoptosis . Additionally, it can affect the expression of genes related to detoxification and stress responses, further influencing cellular metabolism and function .
Molecular Mechanism
The molecular mechanism of 3-(Cystein-S-yl)paracetamol involves its formation through the conjugation of NAPQI with glutathione. This process is catalyzed by glutathione S-transferase, which facilitates the binding of NAPQI to the thiol group of glutathione, forming 3-(Cystein-S-yl)paracetamol . This conjugation reaction is crucial for detoxifying NAPQI and preventing its interaction with cellular proteins and DNA, which can lead to cellular damage and apoptosis .
Furthermore, 3-(Cystein-S-yl)paracetamol can inhibit or activate various enzymes involved in cellular metabolism and detoxification. For example, it can modulate the activity of antioxidant enzymes, such as superoxide dismutase and catalase, thereby influencing the cellular redox state .
Temporal Effects in Laboratory Settings
In laboratory settings, the effects of 3-(Cystein-S-yl)paracetamol can vary over time. Studies have shown that the formation of this compound is time-dependent, with peak levels observed shortly after paracetamol administration . The stability and degradation of 3-(Cystein-S-yl)paracetamol can also influence its long-term effects on cellular function. For instance, prolonged exposure to high levels of this compound can lead to sustained oxidative stress and cellular injury .
Dosage Effects in Animal Models
The effects of 3-(Cystein-S-yl)paracetamol in animal models are dose-dependent. At therapeutic doses, the compound is efficiently detoxified, and no adverse effects are observed . At higher doses, the detoxification pathways can become saturated, leading to the accumulation of NAPQI and subsequent cellular damage . Animal studies have shown that high doses of paracetamol can cause liver and kidney toxicity, with 3-(Cystein-S-yl)paracetamol serving as a biomarker for these toxic effects .
Metabolic Pathways
3-(Cystein-S-yl)paracetamol is involved in several metabolic pathways, primarily related to the detoxification of paracetamol. The compound is formed through the conjugation of NAPQI with glutathione, a process catalyzed by glutathione S-transferase . This pathway is crucial for preventing the accumulation of toxic metabolites and protecting cells from oxidative damage. Additionally, 3-(Cystein-S-yl)paracetamol can influence metabolic flux and metabolite levels by modulating the activity of enzymes involved in cellular metabolism .
Transport and Distribution
The transport and distribution of 3-(Cystein-S-yl)paracetamol within cells and tissues are mediated by various transporters and binding proteins. The compound is primarily transported through the bloodstream to the liver, where it is metabolized and excreted . Within cells, 3-(Cystein-S-yl)paracetamol can interact with transporters such as multidrug resistance-associated proteins (MRPs) and organic anion-transporting polypeptides (OATPs), which facilitate its movement across cellular membranes .
Subcellular Localization
The subcellular localization of 3-(Cystein-S-yl)paracetamol is primarily within the cytoplasm and mitochondria of hepatocytes . The compound can also be found in the nucleus, where it may interact with DNA and other nuclear proteins . The localization of 3-(Cystein-S-yl)paracetamol within specific cellular compartments can influence its activity and function, particularly in terms of its role in detoxification and cellular protection .
Propriétés
IUPAC Name |
(2R)-3-(5-acetamido-2-hydroxyphenyl)sulfanyl-2-aminopropanoic acid | |
---|---|---|
Source | PubChem | |
URL | https://pubchem.ncbi.nlm.nih.gov | |
Description | Data deposited in or computed by PubChem | |
InChI |
InChI=1S/C11H14N2O4S/c1-6(14)13-7-2-3-9(15)10(4-7)18-5-8(12)11(16)17/h2-4,8,15H,5,12H2,1H3,(H,13,14)(H,16,17)/t8-/m0/s1 | |
Source | PubChem | |
URL | https://pubchem.ncbi.nlm.nih.gov | |
Description | Data deposited in or computed by PubChem | |
InChI Key |
LLHICPSCVFRWDT-QMMMGPOBSA-N | |
Source | PubChem | |
URL | https://pubchem.ncbi.nlm.nih.gov | |
Description | Data deposited in or computed by PubChem | |
Canonical SMILES |
CC(=O)NC1=CC(=C(C=C1)O)SCC(C(=O)O)N | |
Source | PubChem | |
URL | https://pubchem.ncbi.nlm.nih.gov | |
Description | Data deposited in or computed by PubChem | |
Isomeric SMILES |
CC(=O)NC1=CC(=C(C=C1)O)SC[C@@H](C(=O)O)N | |
Source | PubChem | |
URL | https://pubchem.ncbi.nlm.nih.gov | |
Description | Data deposited in or computed by PubChem | |
Molecular Formula |
C11H14N2O4S | |
Source | PubChem | |
URL | https://pubchem.ncbi.nlm.nih.gov | |
Description | Data deposited in or computed by PubChem | |
DSSTOX Substance ID |
DTXSID70201608 | |
Record name | 3-(Cystein-S-yl)paracetamol | |
Source | EPA DSSTox | |
URL | https://comptox.epa.gov/dashboard/DTXSID70201608 | |
Description | DSSTox provides a high quality public chemistry resource for supporting improved predictive toxicology. | |
Molecular Weight |
270.31 g/mol | |
Source | PubChem | |
URL | https://pubchem.ncbi.nlm.nih.gov | |
Description | Data deposited in or computed by PubChem | |
CAS No. |
53446-10-9 | |
Record name | Paracetamol-cysteine | |
Source | CAS Common Chemistry | |
URL | https://commonchemistry.cas.org/detail?cas_rn=53446-10-9 | |
Description | CAS Common Chemistry is an open community resource for accessing chemical information. Nearly 500,000 chemical substances from CAS REGISTRY cover areas of community interest, including common and frequently regulated chemicals, and those relevant to high school and undergraduate chemistry classes. This chemical information, curated by our expert scientists, is provided in alignment with our mission as a division of the American Chemical Society. | |
Explanation | The data from CAS Common Chemistry is provided under a CC-BY-NC 4.0 license, unless otherwise stated. | |
Record name | 3-(Cystein-S-yl)paracetamol | |
Source | ChemIDplus | |
URL | https://pubchem.ncbi.nlm.nih.gov/substance/?source=chemidplus&sourceid=0053446109 | |
Description | ChemIDplus is a free, web search system that provides access to the structure and nomenclature authority files used for the identification of chemical substances cited in National Library of Medicine (NLM) databases, including the TOXNET system. | |
Record name | 3-(Cystein-S-yl)paracetamol | |
Source | EPA DSSTox | |
URL | https://comptox.epa.gov/dashboard/DTXSID70201608 | |
Description | DSSTox provides a high quality public chemistry resource for supporting improved predictive toxicology. | |
Record name | 3-CYSTEINYLACETAMINOPHEN | |
Source | FDA Global Substance Registration System (GSRS) | |
URL | https://gsrs.ncats.nih.gov/ginas/app/beta/substances/6Q2X58Y8BP | |
Description | The FDA Global Substance Registration System (GSRS) enables the efficient and accurate exchange of information on what substances are in regulated products. Instead of relying on names, which vary across regulatory domains, countries, and regions, the GSRS knowledge base makes it possible for substances to be defined by standardized, scientific descriptions. | |
Explanation | Unless otherwise noted, the contents of the FDA website (www.fda.gov), both text and graphics, are not copyrighted. They are in the public domain and may be republished, reprinted and otherwise used freely by anyone without the need to obtain permission from FDA. Credit to the U.S. Food and Drug Administration as the source is appreciated but not required. | |
Record name | 3-(Cystein-S-yl)acetaminophen | |
Source | Human Metabolome Database (HMDB) | |
URL | http://www.hmdb.ca/metabolites/HMDB0240217 | |
Description | The Human Metabolome Database (HMDB) is a freely available electronic database containing detailed information about small molecule metabolites found in the human body. | |
Explanation | HMDB is offered to the public as a freely available resource. Use and re-distribution of the data, in whole or in part, for commercial purposes requires explicit permission of the authors and explicit acknowledgment of the source material (HMDB) and the original publication (see the HMDB citing page). We ask that users who download significant portions of the database cite the HMDB paper in any resulting publications. | |
Retrosynthesis Analysis
AI-Powered Synthesis Planning: Our tool employs the Template_relevance Pistachio, Template_relevance Bkms_metabolic, Template_relevance Pistachio_ringbreaker, Template_relevance Reaxys, Template_relevance Reaxys_biocatalysis model, leveraging a vast database of chemical reactions to predict feasible synthetic routes.
One-Step Synthesis Focus: Specifically designed for one-step synthesis, it provides concise and direct routes for your target compounds, streamlining the synthesis process.
Accurate Predictions: Utilizing the extensive PISTACHIO, BKMS_METABOLIC, PISTACHIO_RINGBREAKER, REAXYS, REAXYS_BIOCATALYSIS database, our tool offers high-accuracy predictions, reflecting the latest in chemical research and data.
Strategy Settings
Precursor scoring | Relevance Heuristic |
---|---|
Min. plausibility | 0.01 |
Model | Template_relevance |
Template Set | Pistachio/Bkms_metabolic/Pistachio_ringbreaker/Reaxys/Reaxys_biocatalysis |
Top-N result to add to graph | 6 |
Feasible Synthetic Routes
Q1: What is the significance of quantifying 3-(Cystein-S-yl)paracetamol in paracetamol overdose cases?
A1: 3-(Cystein-S-yl)paracetamol is a metabolite formed when paracetamol undergoes metabolic pathways leading to a toxic byproduct, N-acetyl-p-benzoquinone imine (NAPQI). Quantifying 3-(Cystein-S-yl)paracetamol levels in patients who have overdosed on paracetamol can directly correlate with the extent of liver damage. [] This correlation makes 3-(Cystein-S-yl)paracetamol a potentially valuable biomarker for assessing the severity of paracetamol toxicity and guiding clinical management.
Avertissement et informations sur les produits de recherche in vitro
Veuillez noter que tous les articles et informations sur les produits présentés sur BenchChem sont destinés uniquement à des fins informatives. Les produits disponibles à l'achat sur BenchChem sont spécifiquement conçus pour des études in vitro, qui sont réalisées en dehors des organismes vivants. Les études in vitro, dérivées du terme latin "in verre", impliquent des expériences réalisées dans des environnements de laboratoire contrôlés à l'aide de cellules ou de tissus. Il est important de noter que ces produits ne sont pas classés comme médicaments et n'ont pas reçu l'approbation de la FDA pour la prévention, le traitement ou la guérison de toute condition médicale, affection ou maladie. Nous devons souligner que toute forme d'introduction corporelle de ces produits chez les humains ou les animaux est strictement interdite par la loi. Il est essentiel de respecter ces directives pour assurer la conformité aux normes légales et éthiques en matière de recherche et d'expérimentation.