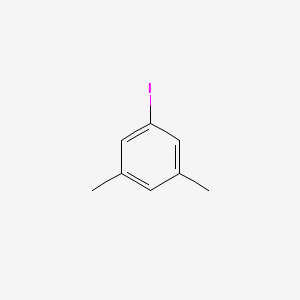
1-Iodo-3,5-dimethylbenzene
Vue d'ensemble
Description
1-Iodo-3,5-dimethylbenzene, also known as 5-iodo-m-xylene, is an aryl halide with the molecular formula C8H9I. It is characterized by the presence of an iodine atom attached to a benzene ring substituted with two methyl groups at the 3 and 5 positions. This compound is a clear light yellow liquid and is sparingly soluble in water .
Mécanisme D'action
Target of Action
1-Iodo-3,5-dimethylbenzene, also known as 5-Iodo-m-xylene, is an aryl halide . Its primary targets are organic compounds that can undergo nucleophilic substitution reactions, such as phenol .
Mode of Action
The compound interacts with its targets through a mechanism known as electrophilic aromatic substitution . In this process, the electrophile (this compound) forms a sigma bond with the benzene ring of the target molecule, generating a positively charged intermediate .
Biochemical Pathways
The affected biochemical pathway involves the copper-catalyzed halogen exchange reaction . This reaction occurs in the presence of NaI or KI in n-BuOH or DMF (solvents) . The downstream effect of this pathway is the formation of new organic compounds, such as 1,3-dimethyl-5-phenoxybenzene .
Pharmacokinetics
The compound’s physical properties, such as its boiling point (92-94 °c/3 mmhg) and density (1608 g/mL at 25 °C), may influence its bioavailability .
Result of Action
The result of the action of this compound is the formation of new organic compounds. For example, it can react with phenol to afford 1,3-dimethyl-5-phenoxybenzene . It is also suitable for use in the synthesis of N-(3,5-xylyl)-N-ethylaniline, an arylamine .
Action Environment
The action of this compound is influenced by environmental factors such as the presence of a catalyst (e.g., CuFe2O4 nano powder) and the type of solvent used (e.g., n-BuOH or DMF) . These factors can significantly affect the compound’s action, efficacy, and stability.
Analyse Biochimique
Biochemical Properties
1-Iodo-3,5-dimethylbenzene plays a significant role in biochemical reactions, particularly in the synthesis of complex organic molecules. It interacts with various enzymes and proteins, facilitating reactions such as α-arylation of ketones and copper-catalyzed N-arylation of imidazoles . These interactions are crucial for the formation of new carbon-carbon and carbon-nitrogen bonds, which are essential in the synthesis of pharmaceuticals and other biologically active compounds.
Cellular Effects
The effects of this compound on cellular processes are diverse. It influences cell function by participating in reactions that modify cellular signaling pathways and gene expression. For instance, its role in the synthesis of N-(3,5-xylyl)-N-ethylaniline can impact cellular metabolism and protein synthesis . Additionally, the compound’s involvement in the formation of 3,5-dimethylbenzonitrile suggests potential effects on cellular detoxification processes.
Molecular Mechanism
At the molecular level, this compound exerts its effects through binding interactions with various biomolecules. It acts as a substrate in enzyme-catalyzed reactions, such as the copper-catalyzed N-arylation of imidazoles . These interactions lead to the formation of new chemical bonds, altering the structure and function of the resulting molecules. The compound’s ability to participate in these reactions highlights its importance in organic synthesis and biochemical research.
Temporal Effects in Laboratory Settings
In laboratory settings, the effects of this compound can change over time due to its stability and degradation. The compound is relatively stable under standard conditions, but its reactivity can be influenced by factors such as temperature and the presence of catalysts . Long-term studies have shown that the compound can maintain its activity in various biochemical reactions, although its effectiveness may decrease over extended periods.
Dosage Effects in Animal Models
The effects of this compound vary with different dosages in animal models. At low doses, the compound can facilitate specific biochemical reactions without causing significant adverse effects. At higher doses, it may exhibit toxic properties, potentially leading to cellular damage and disruption of normal metabolic processes . These dosage-dependent effects are crucial for determining the safe and effective use of the compound in research and therapeutic applications.
Metabolic Pathways
This compound is involved in several metabolic pathways, interacting with enzymes and cofactors to facilitate various biochemical reactions. For example, it participates in the α-arylation of ketones and the copper-catalyzed N-arylation of imidazoles . These interactions can affect metabolic flux and the levels of specific metabolites, highlighting the compound’s role in cellular metabolism and biochemical synthesis.
Transport and Distribution
Within cells and tissues, this compound is transported and distributed through interactions with transporters and binding proteins. These interactions influence the compound’s localization and accumulation, affecting its availability for biochemical reactions . Understanding these transport mechanisms is essential for optimizing the compound’s use in research and therapeutic applications.
Subcellular Localization
The subcellular localization of this compound is determined by targeting signals and post-translational modifications that direct it to specific compartments or organelles. These localization patterns can influence the compound’s activity and function, affecting its role in biochemical reactions and cellular processes
Méthodes De Préparation
1-Iodo-3,5-dimethylbenzene can be synthesized through various methods. One common synthetic route involves the copper-catalyzed halogen exchange reaction. In this method, 5-bromo-m-xylene is reacted with sodium iodide or potassium iodide in solvents such as n-butanol or dimethylformamide . The reaction conditions typically involve heating the mixture to facilitate the exchange of the bromine atom with an iodine atom.
Industrial production methods for this compound are similar to laboratory synthesis but are scaled up to accommodate larger quantities. The use of efficient catalysts and optimized reaction conditions ensures high yield and purity of the final product .
Analyse Des Réactions Chimiques
1-Iodo-3,5-dimethylbenzene undergoes various chemical reactions, including:
Substitution Reactions: It can participate in nucleophilic substitution reactions where the iodine atom is replaced by other nucleophiles. .
Amination: The compound can undergo copper-catalyzed amination to form N-allyl-3,5-dimethylbenzenamine.
Cyanation: It reacts with cyanide sources to form 3,5-dimethylbenzonitrile.
Cross-Coupling Reactions: It is used in Suzuki and other cross-coupling reactions to form various biaryl compounds.
Radical Bromination: The compound can be brominated using N-bromosuccinimide to form 1,3-bis(bromomethyl)-5-iodobenzene.
Common reagents and conditions for these reactions include copper catalysts, phenol, cyanide sources, and N-bromosuccinimide. The major products formed depend on the specific reaction and reagents used.
Applications De Recherche Scientifique
1-Iodo-3,5-dimethylbenzene has several applications in scientific research:
Chemistry: It is used as a starting material in the synthesis of various organic compounds, including arylamines, biaryl compounds, and nitriles
Biology: The compound is used in the synthesis of biologically active molecules and pharmaceuticals.
Medicine: It serves as an intermediate in the production of drugs and other therapeutic agents.
Industry: The compound is employed in the manufacture of specialty chemicals and materials.
Comparaison Avec Des Composés Similaires
1-Iodo-3,5-dimethylbenzene can be compared with other similar compounds, such as:
1-Bromo-3,5-dimethylbenzene: Similar structure but with a bromine atom instead of iodine.
1-Chloro-3,5-dimethylbenzene: Contains a chlorine atom instead of iodine.
The uniqueness of this compound lies in its higher reactivity in substitution reactions compared to its bromo, chloro, and fluoro counterparts, making it a valuable intermediate in organic synthesis.
Propriétés
IUPAC Name |
1-iodo-3,5-dimethylbenzene | |
---|---|---|
Source | PubChem | |
URL | https://pubchem.ncbi.nlm.nih.gov | |
Description | Data deposited in or computed by PubChem | |
InChI |
InChI=1S/C8H9I/c1-6-3-7(2)5-8(9)4-6/h3-5H,1-2H3 | |
Source | PubChem | |
URL | https://pubchem.ncbi.nlm.nih.gov | |
Description | Data deposited in or computed by PubChem | |
InChI Key |
ZLMKEENUYIUKKC-UHFFFAOYSA-N | |
Source | PubChem | |
URL | https://pubchem.ncbi.nlm.nih.gov | |
Description | Data deposited in or computed by PubChem | |
Canonical SMILES |
CC1=CC(=CC(=C1)I)C | |
Source | PubChem | |
URL | https://pubchem.ncbi.nlm.nih.gov | |
Description | Data deposited in or computed by PubChem | |
Molecular Formula |
C8H9I | |
Source | PubChem | |
URL | https://pubchem.ncbi.nlm.nih.gov | |
Description | Data deposited in or computed by PubChem | |
DSSTOX Substance ID |
DTXSID90176985 | |
Record name | Benzene, 1-iodo-3,5-dimethyl- | |
Source | EPA DSSTox | |
URL | https://comptox.epa.gov/dashboard/DTXSID90176985 | |
Description | DSSTox provides a high quality public chemistry resource for supporting improved predictive toxicology. | |
Molecular Weight |
232.06 g/mol | |
Source | PubChem | |
URL | https://pubchem.ncbi.nlm.nih.gov | |
Description | Data deposited in or computed by PubChem | |
CAS No. |
22445-41-6 | |
Record name | Benzene, 1-iodo-3,5-dimethyl- | |
Source | ChemIDplus | |
URL | https://pubchem.ncbi.nlm.nih.gov/substance/?source=chemidplus&sourceid=0022445416 | |
Description | ChemIDplus is a free, web search system that provides access to the structure and nomenclature authority files used for the identification of chemical substances cited in National Library of Medicine (NLM) databases, including the TOXNET system. | |
Record name | Benzene, 1-iodo-3,5-dimethyl- | |
Source | EPA DSSTox | |
URL | https://comptox.epa.gov/dashboard/DTXSID90176985 | |
Description | DSSTox provides a high quality public chemistry resource for supporting improved predictive toxicology. | |
Record name | 5-Iodo-m-xylene | |
Source | European Chemicals Agency (ECHA) | |
URL | https://echa.europa.eu/information-on-chemicals | |
Description | The European Chemicals Agency (ECHA) is an agency of the European Union which is the driving force among regulatory authorities in implementing the EU's groundbreaking chemicals legislation for the benefit of human health and the environment as well as for innovation and competitiveness. | |
Explanation | Use of the information, documents and data from the ECHA website is subject to the terms and conditions of this Legal Notice, and subject to other binding limitations provided for under applicable law, the information, documents and data made available on the ECHA website may be reproduced, distributed and/or used, totally or in part, for non-commercial purposes provided that ECHA is acknowledged as the source: "Source: European Chemicals Agency, http://echa.europa.eu/". Such acknowledgement must be included in each copy of the material. ECHA permits and encourages organisations and individuals to create links to the ECHA website under the following cumulative conditions: Links can only be made to webpages that provide a link to the Legal Notice page. | |
Synthesis routes and methods I
Procedure details
Synthesis routes and methods II
Procedure details
Synthesis routes and methods III
Procedure details
Synthesis routes and methods IV
Procedure details
Retrosynthesis Analysis
AI-Powered Synthesis Planning: Our tool employs the Template_relevance Pistachio, Template_relevance Bkms_metabolic, Template_relevance Pistachio_ringbreaker, Template_relevance Reaxys, Template_relevance Reaxys_biocatalysis model, leveraging a vast database of chemical reactions to predict feasible synthetic routes.
One-Step Synthesis Focus: Specifically designed for one-step synthesis, it provides concise and direct routes for your target compounds, streamlining the synthesis process.
Accurate Predictions: Utilizing the extensive PISTACHIO, BKMS_METABOLIC, PISTACHIO_RINGBREAKER, REAXYS, REAXYS_BIOCATALYSIS database, our tool offers high-accuracy predictions, reflecting the latest in chemical research and data.
Strategy Settings
Precursor scoring | Relevance Heuristic |
---|---|
Min. plausibility | 0.01 |
Model | Template_relevance |
Template Set | Pistachio/Bkms_metabolic/Pistachio_ringbreaker/Reaxys/Reaxys_biocatalysis |
Top-N result to add to graph | 6 |
Feasible Synthetic Routes
Q1: How does the structure of the iron complex influence its reactivity with 1-iodo-3,5-dimethylbenzene?
A1: The research demonstrates that the four-coordinate iron complex [(IPr(2)Me(2))(2)Fe(NHMes)(2)] (where IPr(2)Me(2) = 2,5-diisopropyl-3,4-dimethylimidazol-1-ylidene and Mes = mesityl) reacts with this compound to yield 1-C(6)D(5)-3,5-Me(2)C(6)H(3) and 5-D-1,3-Me(2)C(6)H(3) in deuterated solvents. [] This reactivity is attributed to the steric accessibility of the iron center in the four-coordinate complex. Conversely, the three-coordinate complex [(IPr)Fe(NHDipp)(2)] (where IPr = 2,5-di(2,6-diisopropylphenyl)imidazol-1-ylidene and Dipp = 2,6-diisopropylphenyl) remains inert under similar conditions. This difference highlights the crucial role of steric factors, dictated by the ligand environment around the iron center, in governing the reactivity of these complexes with aryl halides like this compound. []
Avertissement et informations sur les produits de recherche in vitro
Veuillez noter que tous les articles et informations sur les produits présentés sur BenchChem sont destinés uniquement à des fins informatives. Les produits disponibles à l'achat sur BenchChem sont spécifiquement conçus pour des études in vitro, qui sont réalisées en dehors des organismes vivants. Les études in vitro, dérivées du terme latin "in verre", impliquent des expériences réalisées dans des environnements de laboratoire contrôlés à l'aide de cellules ou de tissus. Il est important de noter que ces produits ne sont pas classés comme médicaments et n'ont pas reçu l'approbation de la FDA pour la prévention, le traitement ou la guérison de toute condition médicale, affection ou maladie. Nous devons souligner que toute forme d'introduction corporelle de ces produits chez les humains ou les animaux est strictement interdite par la loi. Il est essentiel de respecter ces directives pour assurer la conformité aux normes légales et éthiques en matière de recherche et d'expérimentation.