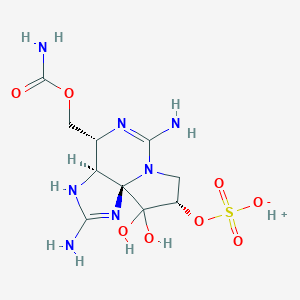
Gonyautoxin III
Vue d'ensemble
Description
Gonyautoxin III is a naturally occurring neurotoxin produced by certain species of marine dinoflagellates, such as Alexandrium, Gonyaulax, and Protogonyaulax . It belongs to the group of saxitoxins, which are known for their potent neurotoxic effects. This compound is one of the eight gonyautoxins identified, and it is known for causing paralytic shellfish poisoning when ingested through contaminated shellfish .
Applications De Recherche Scientifique
Gonyautoxin III has several scientific research applications, including:
Chemistry: It is used as a model compound to study the synthesis and reactivity of neurotoxins.
Biology: Researchers study its effects on marine ecosystems and its role in the food chain.
Medicine: this compound is being investigated for its potential therapeutic applications, such as in the treatment of acute back pain and anal fissures
Mécanisme D'action
Target of Action
Gonyautoxin III, also known as GTX-III or GTX-3, primarily targets sodium channels . These channels play a crucial role in the electrical conduction in cells, particularly in initiating action potentials after the synapse .
Mode of Action
This compound acts as a sodium channel blocker . It binds with high affinity at the site 1 of the α-subunit of the voltage-dependent sodium channels in the postsynaptic membrane . This binding inhibits ion flux through these channels, effectively acting as a “stopper” and disrupting electrical conduction .
Biochemical Pathways
The primary biochemical pathway affected by this compound is the neuronal signaling pathway . By blocking sodium channels, this compound prevents the initiation of action potentials, thereby disrupting neuronal signaling . The downstream effects of this disruption can vary depending on the specific neurons and circuits involved.
Result of Action
The primary result of this compound’s action is the inhibition of electrical conduction in cells . This can lead to various effects depending on the specific cells and tissues involved. For example, in neurons, this can disrupt signaling and potentially lead to symptoms such as paralysis .
Action Environment
The action of this compound can be influenced by various environmental factors. For instance, its production by certain marine dinoflagellate species can lead to its accumulation in shellfish, which can then cause paralytic shellfish poisoning (PSP) in humans when consumed . Therefore, factors such as the presence of these dinoflagellates and the consumption of contaminated shellfish can significantly influence the action, efficacy, and stability of this compound.
Analyse Biochimique
Biochemical Properties
The biochemical properties of Gonyautoxin III are not fully annotated yet . It is known that this compound is part of the group of saxitoxins, which have their structure based on the 2,6-diamino-4-methyl-pyrollo [1,2-c]-purin-10-ol skeleton .
Molecular Mechanism
It is known that gonyautoxins can induce the formation of an antiparallel G-quadruplex structure in aptamer GO18-T-d and combine steadily in the groove at the top of the G-quadruplex structure .
Temporal Effects in Laboratory Settings
It has been shown that gonyautoxins can induce the formation of an antiparallel G-quadruplex structure in aptamer GO18-T-d .
Subcellular Localization
It is known that gonyautoxins can induce the formation of an antiparallel G-quadruplex structure in aptamer GO18-T-d , suggesting that it may localize to the nucleus where DNA is found.
Méthodes De Préparation
Synthetic Routes and Reaction Conditions: The synthesis of Gonyautoxin III involves several steps, starting from L-serine methyl ester. The process includes the formation of a tricyclic core through a rhodium-catalyzed amination reaction. The key steps involve:
- Treatment of L-serine methyl ester with an aldehyde to form a ring structure.
- Deprotection of the formed allyl using a reagent such as SO3CH2CCl3.
- Rhodium-catalyzed amination with guanidine to form the tricyclic frame of this compound .
Industrial Production Methods: The natural production of this compound occurs in marine dinoflagellates, which can be harvested and processed to extract the toxin .
Analyse Des Réactions Chimiques
Types of Reactions: Gonyautoxin III undergoes various chemical reactions, including:
Oxidation: This reaction can modify the functional groups present in the molecule.
Reduction: This reaction can reduce certain functional groups, altering the molecule’s properties.
Substitution: This reaction can replace specific atoms or groups within the molecule with other atoms or groups.
Common Reagents and Conditions:
Oxidation: Reagents such as potassium permanganate or hydrogen peroxide can be used.
Reduction: Reagents such as sodium borohydride or lithium aluminum hydride can be employed.
Substitution: Various nucleophiles or electrophiles can be used depending on the desired substitution.
Major Products Formed: The major products formed from these reactions depend on the specific conditions and reagents used. For example, oxidation may lead to the formation of hydroxylated derivatives, while reduction may yield reduced forms of the toxin .
Comparaison Avec Des Composés Similaires
Saxitoxin: Another potent neurotoxin that shares a similar mechanism of action.
Neosaxitoxin: A derivative of saxitoxin with similar neurotoxic properties.
Decarbamoylsaxitoxin: A variant of saxitoxin with slight structural differences.
Uniqueness of Gonyautoxin III: this compound is unique due to its specific structural features and its ability to epimerize to Gonyautoxin II under certain conditions. This epimerization can affect its potency and toxicity, making it a compound of interest for further research .
Activité Biologique
Gonyautoxin III (GTX3) is a potent marine neurotoxin belonging to the gonyautoxin family, which is associated with paralytic shellfish poisoning (PSP). This article explores the biological activity of GTX3, focusing on its mechanisms of action, toxicity levels, transport mechanisms, and potential therapeutic applications.
Gonyautoxins, including GTX3, exert their effects primarily by binding to voltage-gated sodium channels in excitable cells. This binding inhibits sodium ion influx, leading to a blockade of action potentials in neurons and muscle cells. The result is a significant reduction in neuronal transmission, which can lead to paralysis and respiratory failure in severe cases.
The specific action of GTX3 can be summarized as follows:
- Binding Affinity : GTX3 exhibits a high affinity for sodium channels, comparable to that of saxitoxin (STX), leading to effective inhibition of neuronal activity.
- Reversible Binding : The binding of GTX3 is reversible, allowing for potential recovery if the toxin is removed from the system.
- Toxicity Profile : GTX3 has been shown to have intermediate toxicity compared to other gonyautoxins, with lethal doses causing rapid respiratory failure in animal models .
Toxicity Studies
Research has demonstrated the acute toxicity of GTX3 through various studies. One notable study assessed the median lethal dose (LD50) in mice via intraperitoneal injection:
Toxin | Route of Administration | LD50 (mg/kg) |
---|---|---|
This compound | Intraperitoneal | 0.5 - 1.0 |
Saxitoxin | Intraperitoneal | 0.1 - 0.2 |
Mice exposed to lethal doses exhibited symptoms such as lethargy, irregular breathing, and ultimately death within 20 minutes post-administration . Sublethal doses resulted in a temporary decrease in activity but allowed for recovery within several hours.
Transport Mechanisms
The transport and absorption of GTX3 have been studied using intestinal epithelial cell lines. Research indicates the following:
- Cell Line Studies : In Caco-2 cells, GTX3 showed greater apical-to-basolateral transport compared to the reverse direction, suggesting an active transport mechanism favoring absorption. Conversely, IEC-6 cells exhibited predominant basolateral-to-apical transport .
- Transport Inhibitors : The absorption process was partially Na(+)-dependent and significantly inhibited by adenosine, indicating specific transport pathways involved in GTX3 movement across cell membranes.
Case Studies
A clinical study investigated the application of gonyautoxin 2/3 epimers (which includes GTX3) for therapeutic purposes in reducing anal sphincter tone. Key findings included:
- Study Design : Healthy adults received local injections of GTX2/3 to assess its effect on anal tone.
- Results : All participants showed significant relaxation of the anal sphincter within minutes post-injection, with a marked decrease in maximal voluntary contraction pressure . No adverse effects were reported during or after the study.
Potential Therapeutic Applications
Given its ability to induce muscle relaxation and its reversible binding properties, GTX3 may have potential applications beyond its toxic effects:
Propriétés
IUPAC Name |
[(3aS,4R,9S,10aS)-2,6-diamino-10,10-dihydroxy-9-sulfooxy-3a,4,8,9-tetrahydro-1H-pyrrolo[1,2-c]purin-4-yl]methyl carbamate | |
---|---|---|
Source | PubChem | |
URL | https://pubchem.ncbi.nlm.nih.gov | |
Description | Data deposited in or computed by PubChem | |
InChI |
InChI=1S/C10H17N7O8S/c11-6-15-5-3(2-24-8(13)18)14-7(12)17-1-4(25-26(21,22)23)10(19,20)9(5,17)16-6/h3-5,19-20H,1-2H2,(H2,12,14)(H2,13,18)(H3,11,15,16)(H,21,22,23)/t3-,4-,5-,9-/m0/s1 | |
Source | PubChem | |
URL | https://pubchem.ncbi.nlm.nih.gov | |
Description | Data deposited in or computed by PubChem | |
InChI Key |
ARSXTTJGWGCRRR-LJRZAWCWSA-N | |
Source | PubChem | |
URL | https://pubchem.ncbi.nlm.nih.gov | |
Description | Data deposited in or computed by PubChem | |
Canonical SMILES |
C1C(C(C23N1C(=NC(C2N=C(N3)N)COC(=O)N)N)(O)O)OS(=O)(=O)O | |
Source | PubChem | |
URL | https://pubchem.ncbi.nlm.nih.gov | |
Description | Data deposited in or computed by PubChem | |
Isomeric SMILES |
C1[C@@H](C([C@@]23N1C(=N[C@H]([C@@H]2N=C(N3)N)COC(=O)N)N)(O)O)OS(=O)(=O)O | |
Source | PubChem | |
URL | https://pubchem.ncbi.nlm.nih.gov | |
Description | Data deposited in or computed by PubChem | |
Molecular Formula |
C10H17N7O8S | |
Source | PubChem | |
URL | https://pubchem.ncbi.nlm.nih.gov | |
Description | Data deposited in or computed by PubChem | |
DSSTOX Substance ID |
DTXSID001018107 | |
Record name | Gonyautoxin 3 | |
Source | EPA DSSTox | |
URL | https://comptox.epa.gov/dashboard/DTXSID001018107 | |
Description | DSSTox provides a high quality public chemistry resource for supporting improved predictive toxicology. | |
Molecular Weight |
395.35 g/mol | |
Source | PubChem | |
URL | https://pubchem.ncbi.nlm.nih.gov | |
Description | Data deposited in or computed by PubChem | |
CAS No. |
60537-65-7 | |
Record name | Gonyautoxin III | |
Source | ChemIDplus | |
URL | https://pubchem.ncbi.nlm.nih.gov/substance/?source=chemidplus&sourceid=0060537657 | |
Description | ChemIDplus is a free, web search system that provides access to the structure and nomenclature authority files used for the identification of chemical substances cited in National Library of Medicine (NLM) databases, including the TOXNET system. | |
Record name | Gonyautoxin 3 | |
Source | EPA DSSTox | |
URL | https://comptox.epa.gov/dashboard/DTXSID001018107 | |
Description | DSSTox provides a high quality public chemistry resource for supporting improved predictive toxicology. | |
Record name | 60537-65-7 | |
Source | European Chemicals Agency (ECHA) | |
URL | https://echa.europa.eu/information-on-chemicals | |
Description | The European Chemicals Agency (ECHA) is an agency of the European Union which is the driving force among regulatory authorities in implementing the EU's groundbreaking chemicals legislation for the benefit of human health and the environment as well as for innovation and competitiveness. | |
Explanation | Use of the information, documents and data from the ECHA website is subject to the terms and conditions of this Legal Notice, and subject to other binding limitations provided for under applicable law, the information, documents and data made available on the ECHA website may be reproduced, distributed and/or used, totally or in part, for non-commercial purposes provided that ECHA is acknowledged as the source: "Source: European Chemicals Agency, http://echa.europa.eu/". Such acknowledgement must be included in each copy of the material. ECHA permits and encourages organisations and individuals to create links to the ECHA website under the following cumulative conditions: Links can only be made to webpages that provide a link to the Legal Notice page. | |
Record name | GONYAUTOXIN III | |
Source | FDA Global Substance Registration System (GSRS) | |
URL | https://gsrs.ncats.nih.gov/ginas/app/beta/substances/1FFI43DL2K | |
Description | The FDA Global Substance Registration System (GSRS) enables the efficient and accurate exchange of information on what substances are in regulated products. Instead of relying on names, which vary across regulatory domains, countries, and regions, the GSRS knowledge base makes it possible for substances to be defined by standardized, scientific descriptions. | |
Explanation | Unless otherwise noted, the contents of the FDA website (www.fda.gov), both text and graphics, are not copyrighted. They are in the public domain and may be republished, reprinted and otherwise used freely by anyone without the need to obtain permission from FDA. Credit to the U.S. Food and Drug Administration as the source is appreciated but not required. | |
Retrosynthesis Analysis
AI-Powered Synthesis Planning: Our tool employs the Template_relevance Pistachio, Template_relevance Bkms_metabolic, Template_relevance Pistachio_ringbreaker, Template_relevance Reaxys, Template_relevance Reaxys_biocatalysis model, leveraging a vast database of chemical reactions to predict feasible synthetic routes.
One-Step Synthesis Focus: Specifically designed for one-step synthesis, it provides concise and direct routes for your target compounds, streamlining the synthesis process.
Accurate Predictions: Utilizing the extensive PISTACHIO, BKMS_METABOLIC, PISTACHIO_RINGBREAKER, REAXYS, REAXYS_BIOCATALYSIS database, our tool offers high-accuracy predictions, reflecting the latest in chemical research and data.
Strategy Settings
Precursor scoring | Relevance Heuristic |
---|---|
Min. plausibility | 0.01 |
Model | Template_relevance |
Template Set | Pistachio/Bkms_metabolic/Pistachio_ringbreaker/Reaxys/Reaxys_biocatalysis |
Top-N result to add to graph | 6 |
Feasible Synthetic Routes
Q1: What is the primary mechanism of action for Gonyautoxin 3?
A1: Gonyautoxin 3 (GTX-3) exerts its effects by binding to voltage-gated sodium channels (NaV) in nerve and muscle cells. [, ] This binding effectively blocks the flow of sodium ions, which are crucial for generating and propagating action potentials. [, ] This blockage ultimately leads to paralysis, a hallmark of paralytic shellfish poisoning (PSP).
Q2: Which specific sodium channel isoform does Gonyautoxin 3 exhibit high affinity for?
A2: Research indicates that GTX-3 displays particularly high affinity for the rat NaV1.4 isoform, with an IC50 value of 15 nM. [] This finding suggests a potential role for NaV1.4 in the toxicity of GTX-3.
Q3: Which structural features of Gonyautoxin 3 are crucial for its activity?
A4: While the complete structure-activity relationship of GTX-3 requires further investigation, studies point towards the importance of specific residues in its interaction with sodium channels. For instance, domain III residues T1398 and I1399 in the human Nav1.7 channel play a critical role in the binding affinity of GTX-3. []
Q4: Are there any known instances of Gonyautoxin 3 biotransformation in marine organisms?
A5: Yes, research suggests that scallops can potentially convert ingested PSP toxins into different forms. [] A study analyzing Protogonyaulax tamarensis and scallops from Funka Bay, Hokkaido, found variations in the proportions of GTX-3 and other PSP toxins between the dinoflagellate and the scallops. [] This observation suggests that metabolic processes within the scallop could lead to the transformation of GTX-3 into other related toxins.
Q5: What are the primary Gonyautoxin analogs found in Alexandrium minutum, and how does their toxicity compare?
A6: Analysis of Alexandrium minutum strains reveals the presence of Gonyautoxin-1, Gonyautoxin-2, Gonyautoxin-3, and Gonyautoxin-4. [] Notably, the Amtk4 strain of A. minutum exhibits a significantly higher total gonyautoxin content compared to the Amtk2 strain. [] This difference highlights the variability in toxicity even within a single species.
Q6: What is the toxin profile of Alexandrium tamarense and how does it vary between strains?
A7: Alexandrium tamarense strains exhibit varying toxin profiles. For instance, strain HK9301 primarily contains Gonyautoxin-2 and Gonyautoxin-3, while strain CI01 lacks detectable levels of these toxins. [] This difference emphasizes the importance of strain-specific analysis when assessing the potential risks associated with A. tamarense blooms.
Q7: Have any novel Gonyautoxin analogs been identified in recent years?
A8: Research on Gymnodinium catenatum from the Portuguese coast identified two new presumed N-hydroxylated analogs of G. catenatum toxins GC2 and GC3. [] These new analogs, designated GC5 and GC6, contribute to the expanding knowledge of emerging PST classes. []
Q8: How stable is Gonyautoxin 3 under different conditions?
A9: While specific data on the stability of GTX-3 under various conditions is limited in the provided abstracts, studies on related PSP toxins offer some insights. For example, a mixture of Gonyautoxin 2 and Gonyautoxin 3, when heated in water at various temperatures, showed significant degradation, with less than 3% of initial toxicity remaining after heating at 120°C for 180 minutes. [] This finding indicates the potential for thermal degradation of GTX-3 and possibly other related toxins.
Q9: What analytical techniques are commonly employed to detect and quantify Gonyautoxin 3?
A10: High-performance liquid chromatography (HPLC) coupled with fluorescence detection is a commonly employed method for analyzing PSP toxins, including GTX-3. [, , , ] Additionally, High-Performance Liquid Chromatography Tandem Mass Spectrometry (HILIC-MS/MS) has been used to identify known and emerging PSTs in Gymnodinium catenatum. [] Cellulose acetate membrane electrophoresis can also be utilized for analysis. []
Avertissement et informations sur les produits de recherche in vitro
Veuillez noter que tous les articles et informations sur les produits présentés sur BenchChem sont destinés uniquement à des fins informatives. Les produits disponibles à l'achat sur BenchChem sont spécifiquement conçus pour des études in vitro, qui sont réalisées en dehors des organismes vivants. Les études in vitro, dérivées du terme latin "in verre", impliquent des expériences réalisées dans des environnements de laboratoire contrôlés à l'aide de cellules ou de tissus. Il est important de noter que ces produits ne sont pas classés comme médicaments et n'ont pas reçu l'approbation de la FDA pour la prévention, le traitement ou la guérison de toute condition médicale, affection ou maladie. Nous devons souligner que toute forme d'introduction corporelle de ces produits chez les humains ou les animaux est strictement interdite par la loi. Il est essentiel de respecter ces directives pour assurer la conformité aux normes légales et éthiques en matière de recherche et d'expérimentation.