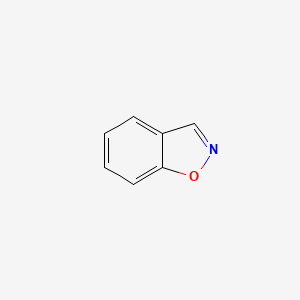
1,2-Benzisoxazole
Vue d'ensemble
Description
1,2-Benzisoxazole is an aromatic organic compound with the molecular formula C₇H₅NO. It features a benzene ring fused to an isoxazole ring, forming a heterocyclic structure. This compound is notable for its stability due to its aromaticity, although it is only weakly basic. While this compound itself does not have common applications, its derivatives are widely used in pharmaceuticals, including antipsychotic drugs such as risperidone and paliperidone, and the anticonvulsant zonisamide .
Mécanisme D'action
Target of Action
1,2-Benzisoxazole has been found to interact with various targets. It has shown antibiotic activity against multi-drug resistant Acinetobacter baumannii . It also interacts with enzymes such as chorismate pyruvate-lyase and 4-hydroxybenzoate octaprenyl-transferase . In the context of antipsychotic drugs, it has been found to block dopamine D2 receptors in the mesolimbic region .
Mode of Action
The mode of action of this compound varies depending on the target. For instance, in its role as an antibiotic, it inhibits the growth of Acinetobacter baumannii . As an antipsychotic, it blocks dopamine D2 receptors, which are associated with increased dopaminergic activity .
Biochemical Pathways
This compound affects various biochemical pathways. In the case of Acinetobacter baumannii, it interferes with the metabolic pathways involving 4-hydroxybenzoate . In the context of antipsychotics, it influences the dopaminergic pathways in the mesolimbic region .
Pharmacokinetics
Zonisamide, a synthetic this compound-3-methanesulfonamide, is known to have a long half-life (63-69h in healthy volunteers), allowing for once or twice-daily dosing . Since zonisamide is metabolized by cytochrome p450, liver enzyme-inducing antiepileptic drugs will increase zonisamide clearance, and dosage adjustments may be necessary when it is used in combination with certain antiepileptic drugs .
Result of Action
The result of this compound’s action depends on its target. As an antibiotic, it inhibits the growth of Acinetobacter baumannii . As an antipsychotic, it reduces symptoms associated with increased dopaminergic activity .
Action Environment
The action of this compound can be influenced by environmental factors. For instance, the presence of 4-hydroxybenzoate in minimal media was able to reverse this compound’s antibacterial effects in A. baumannii
Analyse Biochimique
Biochemical Properties
1,2-Benzisoxazole plays a crucial role in various biochemical reactions. It interacts with several enzymes, proteins, and other biomolecules. For instance, it has been identified to interact with chorismate pyruvate-lyase and 4-hydroxybenzoate octaprenyl-transferase, which are involved in metabolic pathways . These interactions are essential for its antibacterial activity against multi-drug resistant pathogens like Acinetobacter baumannii .
Cellular Effects
This compound influences various cellular processes and functions. It has been shown to affect cell signaling pathways, gene expression, and cellular metabolism. For example, its antibacterial properties are linked to its ability to disrupt the metabolic processes of pathogenic bacteria, leading to cell death . Additionally, this compound exhibits anticancer properties by inducing apoptosis and inhibiting cell proliferation in cancer cells .
Molecular Mechanism
The molecular mechanism of this compound involves binding interactions with specific biomolecules, enzyme inhibition, and changes in gene expression. It binds to enzymes like chorismate pyruvate-lyase, inhibiting their activity and disrupting essential metabolic pathways in bacteria . This inhibition leads to the accumulation of toxic intermediates, ultimately causing bacterial cell death. In cancer cells, this compound modulates gene expression to induce apoptosis and inhibit cell growth .
Temporal Effects in Laboratory Settings
In laboratory settings, the effects of this compound change over time. The compound’s stability and degradation are critical factors influencing its long-term effects on cellular function. Studies have shown that this compound remains stable under specific conditions, maintaining its antibacterial and anticancer activities over extended periods
Dosage Effects in Animal Models
The effects of this compound vary with different dosages in animal models. At lower doses, it exhibits therapeutic effects without significant toxicity. At higher doses, toxic or adverse effects may occur. For instance, high doses of this compound can lead to hepatotoxicity and nephrotoxicity in animal models . Therefore, determining the optimal dosage is crucial for maximizing its therapeutic benefits while minimizing potential risks.
Metabolic Pathways
This compound is involved in several metabolic pathways, interacting with enzymes and cofactors. It affects metabolic flux and metabolite levels, influencing the overall metabolic state of cells. For example, its interaction with chorismate pyruvate-lyase and 4-hydroxybenzoate octaprenyl-transferase disrupts the synthesis of essential metabolites in bacteria, leading to their antibacterial effects . Additionally, this compound may modulate metabolic pathways in cancer cells, contributing to its anticancer properties .
Transport and Distribution
Within cells and tissues, this compound is transported and distributed through specific transporters and binding proteins. These interactions influence its localization and accumulation, affecting its biological activity. For instance, the compound’s distribution in bacterial cells is crucial for its antibacterial effects, as it needs to reach its target enzymes to exert its inhibitory action . Similarly, its distribution in cancer cells determines its efficacy in inducing apoptosis and inhibiting cell proliferation .
Subcellular Localization
The subcellular localization of this compound is essential for its activity and function. It may be directed to specific compartments or organelles through targeting signals or post-translational modifications. In bacteria, this compound localizes to the cytoplasm, where it interacts with target enzymes . In cancer cells, its subcellular localization may influence its ability to modulate gene expression and induce apoptosis .
Méthodes De Préparation
1,2-Benzisoxazole can be synthesized through various methods. One common synthetic route involves the base-catalyzed reaction of salicylaldehyde with hydroxylamine-O-sulfonic acid at room temperature . Another method includes the oxidative cyclization of o-acylphenol oximes using lead (IV) acetate or sodium hypochlorite . These methods are efficient and provide good yields of the desired product.
In industrial settings, the synthesis of this compound derivatives often involves similar routes but on a larger scale, with careful control of reaction conditions to ensure high purity and yield. The choice of reagents and catalysts can vary depending on the specific derivative being produced and the desired properties of the final product.
Analyse Des Réactions Chimiques
1,2-Benzisoxazole undergoes various chemical reactions, including:
Oxidation: The compound can be oxidized to form this compound 2-oxides.
Substitution: Nitration and bromination are common substitution reactions for this compound.
Kemp Elimination: This reaction involves the cleavage of the relatively weak N-O bond by a strong base, yielding a 2-hydroxybenzonitrile species.
The major products formed from these reactions depend on the specific reagents and conditions used. For example, nitration typically results in nitro-substituted benzisoxazoles, while oxidation yields benzisoxazole 2-oxides.
Applications De Recherche Scientifique
Comparaison Avec Des Composés Similaires
1,2-Benzisoxazole is similar to other heterocyclic compounds such as benzoxazole and anthranil. These compounds share a fused benzene ring structure but differ in the heteroatoms present in the ring:
Benzoxazole: Contains an oxygen atom in place of the nitrogen atom found in benzisoxazole.
Anthranil: Features a nitrogen atom in the ring but lacks the oxygen atom present in benzisoxazole.
The unique combination of nitrogen and oxygen in the isoxazole ring of this compound imparts distinct chemical properties and reactivity, making it valuable in various applications.
Activité Biologique
1,2-Benzisoxazole is a heterocyclic compound that has garnered significant attention in medicinal chemistry due to its diverse biological activities. This article explores the biological activity of this compound, highlighting its antibacterial, anti-inflammatory, anticonvulsant, and anticancer properties. The synthesis of various derivatives and their corresponding biological effects are also discussed.
Overview of Biological Activities
This compound and its derivatives exhibit a wide range of biological activities:
- Antibacterial Activity : this compound has shown potent antibacterial effects against various pathogens, particularly multi-drug resistant strains of Acinetobacter baumannii with minimum inhibitory concentrations (MIC) as low as 6.25 μg/mL . The mechanism of action is believed to involve the inhibition of specific metabolic pathways in bacteria.
- Anti-inflammatory Effects : Certain derivatives have demonstrated significant anti-inflammatory properties, making them potential candidates for treating inflammatory diseases .
- Anticonvulsant Activity : Research indicates that some this compound derivatives possess anticonvulsant properties, with specific substitutions enhancing their activity .
- Anticancer Properties : Compounds within this class have been investigated for their potential anticancer effects, showing promise in inhibiting tumor growth .
Structure-Activity Relationship (SAR)
The biological activity of this compound is heavily influenced by its structural modifications. A few notable findings include:
- Substituents on the Phenyl Ring : Compounds bearing activating groups such as methyl or methoxy exhibited enhanced antibacterial and antioxidant activities. For instance, a derivative with a methoxy group displayed an MIC of 3.25 μg/mL against E. coli .
- Halogen Substitution : The introduction of halogen atoms at specific positions on the benzisoxazole ring has been shown to increase both activity and neurotoxicity .
Case Study 1: Antibacterial Efficacy Against A. baumannii
A study identified a naturally occurring this compound produced by Bradyrhizobium denitrificans, which demonstrated significant antibacterial activity against clinical strains of A. baumannii. The compound's effectiveness was linked to its ability to target bacterial metabolic processes involving 4-hydroxybenzoate .
Compound | MIC (μg/mL) | Target Pathogen |
---|---|---|
3,6-Dihydroxy-1,2-benzisoxazole | 6.25 | Acinetobacter baumannii |
Zonisamide | Varies | Epilepsy-related strains |
Case Study 2: Anticonvulsant Activity
Research on 3-substituted this compound derivatives revealed marked anticonvulsant activity in mice. The most promising compound was identified as 3-(sulfamoylmethyl)-1,2-benzisoxazole, showing a favorable ratio of neurotoxicity to efficacy .
Synthesis and Derivatives
The synthesis of this compound can be achieved through various methods, including cyclization reactions involving salicylaldoximes. Recent studies have focused on creating novel derivatives that enhance biological activity while reducing toxicity.
Propriétés
IUPAC Name |
1,2-benzoxazole | |
---|---|---|
Source | PubChem | |
URL | https://pubchem.ncbi.nlm.nih.gov | |
Description | Data deposited in or computed by PubChem | |
InChI |
InChI=1S/C7H5NO/c1-2-4-7-6(3-1)5-8-9-7/h1-5H | |
Source | PubChem | |
URL | https://pubchem.ncbi.nlm.nih.gov | |
Description | Data deposited in or computed by PubChem | |
InChI Key |
KTZQTRPPVKQPFO-UHFFFAOYSA-N | |
Source | PubChem | |
URL | https://pubchem.ncbi.nlm.nih.gov | |
Description | Data deposited in or computed by PubChem | |
Canonical SMILES |
C1=CC=C2C(=C1)C=NO2 | |
Source | PubChem | |
URL | https://pubchem.ncbi.nlm.nih.gov | |
Description | Data deposited in or computed by PubChem | |
Molecular Formula |
C7H5NO | |
Source | PubChem | |
URL | https://pubchem.ncbi.nlm.nih.gov | |
Description | Data deposited in or computed by PubChem | |
DSSTOX Substance ID |
DTXSID20181567 | |
Record name | Benz(d)isoxazole | |
Source | EPA DSSTox | |
URL | https://comptox.epa.gov/dashboard/DTXSID20181567 | |
Description | DSSTox provides a high quality public chemistry resource for supporting improved predictive toxicology. | |
Molecular Weight |
119.12 g/mol | |
Source | PubChem | |
URL | https://pubchem.ncbi.nlm.nih.gov | |
Description | Data deposited in or computed by PubChem | |
CAS No. |
271-95-4 | |
Record name | 1,2-Benzisoxazole | |
Source | CAS Common Chemistry | |
URL | https://commonchemistry.cas.org/detail?cas_rn=271-95-4 | |
Description | CAS Common Chemistry is an open community resource for accessing chemical information. Nearly 500,000 chemical substances from CAS REGISTRY cover areas of community interest, including common and frequently regulated chemicals, and those relevant to high school and undergraduate chemistry classes. This chemical information, curated by our expert scientists, is provided in alignment with our mission as a division of the American Chemical Society. | |
Explanation | The data from CAS Common Chemistry is provided under a CC-BY-NC 4.0 license, unless otherwise stated. | |
Record name | Indoxazene | |
Source | ChemIDplus | |
URL | https://pubchem.ncbi.nlm.nih.gov/substance/?source=chemidplus&sourceid=0000271954 | |
Description | ChemIDplus is a free, web search system that provides access to the structure and nomenclature authority files used for the identification of chemical substances cited in National Library of Medicine (NLM) databases, including the TOXNET system. | |
Record name | Benz(d)isoxazole | |
Source | EPA DSSTox | |
URL | https://comptox.epa.gov/dashboard/DTXSID20181567 | |
Description | DSSTox provides a high quality public chemistry resource for supporting improved predictive toxicology. | |
Record name | Benz[d]isoxazole | |
Source | European Chemicals Agency (ECHA) | |
URL | https://echa.europa.eu/substance-information/-/substanceinfo/100.005.440 | |
Description | The European Chemicals Agency (ECHA) is an agency of the European Union which is the driving force among regulatory authorities in implementing the EU's groundbreaking chemicals legislation for the benefit of human health and the environment as well as for innovation and competitiveness. | |
Explanation | Use of the information, documents and data from the ECHA website is subject to the terms and conditions of this Legal Notice, and subject to other binding limitations provided for under applicable law, the information, documents and data made available on the ECHA website may be reproduced, distributed and/or used, totally or in part, for non-commercial purposes provided that ECHA is acknowledged as the source: "Source: European Chemicals Agency, http://echa.europa.eu/". Such acknowledgement must be included in each copy of the material. ECHA permits and encourages organisations and individuals to create links to the ECHA website under the following cumulative conditions: Links can only be made to webpages that provide a link to the Legal Notice page. | |
Record name | INDOXAZENE | |
Source | FDA Global Substance Registration System (GSRS) | |
URL | https://gsrs.ncats.nih.gov/ginas/app/beta/substances/D879RKM5NQ | |
Description | The FDA Global Substance Registration System (GSRS) enables the efficient and accurate exchange of information on what substances are in regulated products. Instead of relying on names, which vary across regulatory domains, countries, and regions, the GSRS knowledge base makes it possible for substances to be defined by standardized, scientific descriptions. | |
Explanation | Unless otherwise noted, the contents of the FDA website (www.fda.gov), both text and graphics, are not copyrighted. They are in the public domain and may be republished, reprinted and otherwise used freely by anyone without the need to obtain permission from FDA. Credit to the U.S. Food and Drug Administration as the source is appreciated but not required. | |
Synthesis routes and methods I
Procedure details
Synthesis routes and methods II
Procedure details
Synthesis routes and methods III
Procedure details
Synthesis routes and methods IV
Procedure details
Synthesis routes and methods V
Procedure details
Retrosynthesis Analysis
AI-Powered Synthesis Planning: Our tool employs the Template_relevance Pistachio, Template_relevance Bkms_metabolic, Template_relevance Pistachio_ringbreaker, Template_relevance Reaxys, Template_relevance Reaxys_biocatalysis model, leveraging a vast database of chemical reactions to predict feasible synthetic routes.
One-Step Synthesis Focus: Specifically designed for one-step synthesis, it provides concise and direct routes for your target compounds, streamlining the synthesis process.
Accurate Predictions: Utilizing the extensive PISTACHIO, BKMS_METABOLIC, PISTACHIO_RINGBREAKER, REAXYS, REAXYS_BIOCATALYSIS database, our tool offers high-accuracy predictions, reflecting the latest in chemical research and data.
Strategy Settings
Precursor scoring | Relevance Heuristic |
---|---|
Min. plausibility | 0.01 |
Model | Template_relevance |
Template Set | Pistachio/Bkms_metabolic/Pistachio_ringbreaker/Reaxys/Reaxys_biocatalysis |
Top-N result to add to graph | 6 |
Feasible Synthetic Routes
Q1: What is the molecular formula and weight of 1,2-benzisoxazole?
A1: The molecular formula of this compound is C7H5NO, and its molecular weight is 119.12 g/mol.
Q2: How can this compound derivatives be characterized spectroscopically?
A2: Common spectroscopic techniques used for characterizing this compound derivatives include:
- Infrared (IR) Spectroscopy: Identifies functional groups through characteristic absorption bands, such as C=N stretching, C-O stretching, and aromatic C-H stretching. [, , , ]
- Nuclear Magnetic Resonance (NMR) Spectroscopy: Provides information about the structure, including the number and types of hydrogen and carbon atoms, as well as their connectivity. Both 1H NMR and 13C NMR are commonly employed. [, , , , , , , , , ]
- Mass Spectrometry (MS): Determines the molecular weight and fragmentation pattern, aiding in structure elucidation. Techniques like FAB-MS are frequently used. [, , , , , ]
Q3: What are some common synthetic approaches to 1,2-benzisoxazoles?
A3: Several methods have been reported for synthesizing 1,2-benzisoxazoles, including:
- Cyclization of o-hydroxyaryl ketoximes: This approach, often mediated by polyphosphoric acid or other dehydrating agents, is widely employed. [, ]
- Modified Boekelheide Rearrangement: This method involves the conversion of this compound N-oxides to 3-chloromethyl-1,2-benzisoxazoles using phosphorus oxychloride in the presence of organic bases. []
- TBAF-Mediated 1,3-Dipolar Cycloaddition: This approach involves the in situ generation of nitrile oxides and benzyne, which rapidly react to form 1,2-benzisoxazoles in good yields. []
Q4: How does the substituent at the 3-position influence the reactivity of 1,2-benzisoxazoles?
A4: The substituent at the 3-position plays a crucial role in the reactivity and biological activity of 1,2-benzisoxazoles. For example, 3-amino-1,2-benzisoxazoles can be synthesized via a novel route involving 2-[(isopropylideneamino)oxy]benzonitrile, offering a pathway to diverse isoxazolo[3,4,5-ef][1,4]benzoxazepine ring systems. []
Q5: What types of reactions are this compound 2-oxides known to undergo?
A5: Research indicates that while nitration of this compound 2-oxides proceeds via simple substitution, other reactions, such as bromination, treatment with acetic anhydride, halogen acids, or sodium alkoxide, typically result in the cleavage of the heterocyclic ring. [, ]
Q6: What are the primary therapeutic applications of this compound derivatives?
A6: this compound derivatives exhibit a broad spectrum of biological activities, making them attractive targets for drug discovery. They are primarily recognized for their potential in treating CNS disorders:
- Anticonvulsant Activity: Derivatives like 3-(sulfamoylmethyl)-1,2-benzisoxazole (Zonisamide) act as anticonvulsant agents, potentially by blocking voltage-sensitive sodium channels and reducing T-type calcium currents. [, , ]
- Antipsychotic Activity: Several 1,2-benzisoxazoles, including Risperidone, Paliperidone, and Iloperidone, are clinically used atypical antipsychotics. []
Q7: How does the structure of this compound contribute to its designation as a "privileged structure" in medicinal chemistry?
A7: this compound is considered a "privileged structure" due to its ability to interact with a diverse range of biological targets, particularly within the CNS. This versatility arises from its unique structural features:
- Presence of Polar Groups: The nitrogen and oxygen atoms within the ring, as well as the potential for introducing various substituents, allow for hydrogen bonding and other polar interactions with target proteins. []
Q8: How do structural modifications of 1,2-benzisoxazoles influence their anticonvulsant activity?
A8: Structure-activity relationship (SAR) studies on 3-(sulfamoylmethyl)-1,2-benzisoxazole analogues have revealed key insights:
- Halogenation: Introduction of a halogen atom at the 5-position of the benzisoxazole ring often enhances anticonvulsant activity but may also increase neurotoxicity. []
- Sulfamoyl Group Modification: Modifications to the sulfamoyl group tend to decrease anticonvulsant activity. []
- Alkylation: The activity of monoalkylated compounds might be influenced by metabolic biotransformation. []
Q9: Can you provide examples of how specific substituents on the this compound core affect biological activity?
A9: Certainly, here are a few examples:
- Neuroleptic Activity: Studies on 3-(1-substituted-4-piperidinyl)-1,2-benzisoxazoles revealed that a 6-fluoro substituent on the benzisoxazole ring, along with a (1,3-dihydro-2-oxo-2H-benzimidazol-1-yl)propyl group at the piperidine nitrogen, led to enhanced potency in climbing mice assays and [3H]spiroperidol binding assays. []
- Cholinesterase Inhibition: In a series of 3-(4-substituted-1-piperidinyl)-6-halo-1,2-benzisoxazole hydrochlorides, a 6-fluoro substituent on the benzisoxazole ring, combined with a 1-morpholine hydrochloride substituent, resulted in the most potent inhibition of acetylcholinesterase. []
Q10: What are the primary metabolic pathways involved in the breakdown of zonisamide?
A10: Zonisamide is primarily metabolized through reductive and conjugative pathways. [] Key steps include:
- Reductive Cleavage: The this compound ring undergoes reductive cleavage, primarily catalyzed by aldehyde oxidase, a cytosolic enzyme. This reaction leads to the formation of 2-sulfamoylacetylphenol, a major metabolite. [, ]
- Conjugation: Both zonisamide and its metabolites can undergo conjugation reactions, such as glucuronidation, to increase water solubility and facilitate excretion. []
Q11: Are there significant species differences in the metabolism of zonisamide?
A11: Yes, studies have shown interspecies variability in zonisamide metabolism. For example:
- Aldehyde Oxidase Activity: The activity of aldehyde oxidase, a key enzyme involved in zonisamide's reductive metabolism, varies significantly across species. Rabbits, hamsters, mice, and guinea pigs exhibit higher cytosolic aldehyde oxidase activity compared to rats. []
Q12: How has computational chemistry been employed to study this compound derivatives?
A12: Computational tools have proven valuable in understanding and predicting the properties and activities of 1,2-benzisoxazoles:
- Molecular Docking: This technique helps predict the binding modes and affinities of this compound derivatives to target proteins. Studies have utilized molecular docking to assess analgesic and anti-inflammatory activities by targeting nicotinic acetylcholine receptors and COX-2, respectively. []
- QSAR Modeling: Quantitative structure-activity relationship (QSAR) models, often derived from computational calculations and experimental data, can correlate structural features of 1,2-benzisoxazoles with their biological activities. These models aid in designing new derivatives with improved potency and selectivity. []
Avertissement et informations sur les produits de recherche in vitro
Veuillez noter que tous les articles et informations sur les produits présentés sur BenchChem sont destinés uniquement à des fins informatives. Les produits disponibles à l'achat sur BenchChem sont spécifiquement conçus pour des études in vitro, qui sont réalisées en dehors des organismes vivants. Les études in vitro, dérivées du terme latin "in verre", impliquent des expériences réalisées dans des environnements de laboratoire contrôlés à l'aide de cellules ou de tissus. Il est important de noter que ces produits ne sont pas classés comme médicaments et n'ont pas reçu l'approbation de la FDA pour la prévention, le traitement ou la guérison de toute condition médicale, affection ou maladie. Nous devons souligner que toute forme d'introduction corporelle de ces produits chez les humains ou les animaux est strictement interdite par la loi. Il est essentiel de respecter ces directives pour assurer la conformité aux normes légales et éthiques en matière de recherche et d'expérimentation.