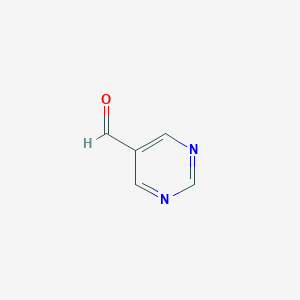
Pyrimidine-5-carbaldehyde
Vue d'ensemble
Description
Pyrimidine-5-carbaldehyde (CAS: 10070-92-5) is an aromatic aldehyde with the molecular formula C₅H₄N₂O and a molecular weight of 108.10 g/mol. It is characterized by a pyrimidine ring substituted with a formyl group at the 5-position. This compound serves as a critical intermediate in organic synthesis, particularly in asymmetric autocatalysis and the preparation of chiral pharmaceuticals or agrochemicals . For example, it is used to synthesize mesoionic insecticide intermediates via condensation reactions with amines . Its reactivity with organozinc reagents (e.g., diisopropylzinc, i-Pr₂Zn) enables enantioselective C–C bond formation, a cornerstone of the Soai reaction, which amplifies enantiomeric excess (ee) without external chiral sources .
This compound exhibits unique dynamic behavior in solution. In the presence of alcohols like 2-propanol, it forms hemiacetals (e.g., (R)-5iPr or (S)-5iPr), as observed via HPLC with chiral stationary phases . Kinetic studies reveal that hemiacetal formation is endergonic (ΔH‡ = 26.3 ± 0.2 kJ/mol) and highly dynamic, with a reaction rate constant of 4.1 × 10⁻³ (mol × s)⁻¹ at 293 K .
Méthodes De Préparation
Synthetic Routes and Reaction Conditions: One common method for synthesizing pyrimidine-5-carbaldehyde involves the reaction of 5-bromopyrimidine with n-butyllithium in anhydrous tetrahydrofuran (THF) at -78°C, followed by the addition of ethyl formate. The reaction mixture is then quenched with hydrochloric acid and purified via flash column chromatography .
Industrial Production Methods: Industrial production methods for this compound typically involve similar synthetic routes but on a larger scale. The use of automated reactors and continuous flow systems can enhance the efficiency and yield of the production process. The reaction conditions are carefully controlled to ensure the purity and quality of the final product .
Analyse Des Réactions Chimiques
Types of Reactions: Pyrimidine-5-carbaldehyde undergoes various chemical reactions, including:
Oxidation: The aldehyde group can be oxidized to form pyrimidine-5-carboxylic acid.
Reduction: The aldehyde group can be reduced to form pyrimidine-5-methanol.
Substitution: The compound can undergo nucleophilic substitution reactions, particularly at the 2- and 4-positions of the pyrimidine ring.
Common Reagents and Conditions:
Oxidation: Common oxidizing agents include potassium permanganate and chromium trioxide.
Reduction: Common reducing agents include sodium borohydride and lithium aluminum hydride.
Substitution: Reagents such as Grignard reagents and organolithium compounds are commonly used.
Major Products:
Oxidation: Pyrimidine-5-carboxylic acid.
Reduction: Pyrimidine-5-methanol.
Substitution: Various substituted pyrimidine derivatives depending on the nucleophile used.
Applications De Recherche Scientifique
Organic Synthesis
Pyrimidine-5-carbaldehyde serves as a key intermediate in the synthesis of various biologically active compounds. It is particularly useful in the preparation of pyrimidyl alkanols through reactions with organometallic reagents such as diisopropylzinc. This reaction leads to the formation of both (S)- and (R)-pyrimidyl alkanols, which are valuable for further chemical transformations .
Table 1: Reactions Involving this compound
Reaction Type | Reagents Used | Products Formed |
---|---|---|
Grignard Reaction | Diisopropylzinc | (S)- and (R)-pyrimidyl alkanols |
Condensation | Amines | Schiff bases |
Cyclization | Various aldehydes | Pyrimidine derivatives |
Medicinal Chemistry
Pyrimidine derivatives have shown significant promise in medicinal chemistry, particularly as potential antibacterial and anticancer agents. For instance, recent studies have synthesized Schiff bases from this compound that exhibit selective antibacterial activity against Enterococcus faecalis, a common nosocomial pathogen. One such compound demonstrated superior anticancer properties against gastric adenocarcinoma cells compared to its analogs .
Case Study: Antibacterial Activity
In a study conducted by Al Neyadi et al., a new Schiff base derived from this compound was evaluated for its antibacterial properties. The compound exhibited strong activity against E. faecalis, highlighting the potential of pyrimidine derivatives in combating antibiotic-resistant bacteria .
Biological Research
This compound is also utilized in biological research for the development of enzyme inhibitors. For example, modifications to the pyrimidine ring structure have led to the discovery of potent inhibitors of pyruvate dehydrogenase multienzyme complex E1 (PDHc E1), which is crucial for cellular metabolism. These compounds showed bactericidal activity with minimal toxicity to mammalian cells, making them suitable candidates for further optimization .
Table 2: Biological Activities of Pyrimidine Derivatives
Compound Name | Activity Type | Target Organism/Cell Type |
---|---|---|
5-[(4-ethoxyphenyl)imino]methyl-N-(4-fluorophenyl)-6-methyl-2-phenylpyrimidin-4-amine | Antibacterial | E. faecalis |
Various pyrimidine derivatives | Anticancer | Gastric adenocarcinoma cells |
Pyruvate dehydrogenase inhibitors | Enzyme inhibition | E. coli PDHc E1 |
Applications in Asymmetric Synthesis
This compound has been employed as a starting material in asymmetric autocatalysis, showcasing its utility in producing enantiomerically enriched compounds. This application is particularly relevant in developing pharmaceuticals where chirality plays a critical role in drug efficacy and safety .
Mécanisme D'action
The mechanism of action of pyrimidine-5-carbaldehyde is primarily related to its ability to participate in various chemical reactions due to the presence of the aldehyde group. This functional group can form covalent bonds with nucleophiles, leading to the formation of new compounds. In biological systems, pyrimidine derivatives can interact with enzymes and nucleic acids, influencing various biochemical pathways .
Comparaison Avec Des Composés Similaires
Comparison with Structurally Similar Compounds
Pyrimidine-5-carbaldehyde derivatives and analogs vary in substituents and electronic properties, leading to distinct reactivities and applications. Below is a detailed comparison:
Substituted Pyrimidine Carbaldehydes
Key Findings:
- Steric Effects: Bulky groups like morpholine (in 2-morpholino derivatives) may hinder coordination with organozinc reagents, altering enantioselectivity in autocatalytic reactions .
- Amino/Methyl Substitution: Amino groups (e.g., in 5-amino-2-methyl derivatives) introduce hydrogen-bonding capability, expanding utility in supramolecular chemistry .
Reactivity in Asymmetric Autocatalysis
This compound is central to the Soai reaction, where it reacts with i-Pr₂Zn to form enantioenriched 5-pyrimidyl alkanols (>99.5% ee) without added chiral agents . In contrast, analogs like 2-(tert-butyldimethylsilylethynyl)this compound exhibit modified reactivity due to propargyl substituents, which stabilize transition states via π-interactions but require chiral mesoporous silica catalysts for high ee .
Critical Differences:
- Autocatalytic Amplification : Only this compound demonstrates spontaneous symmetry breaking in the Soai reaction, whereas substituted analogs rely on external chiral initiators (e.g., chiral crystals, deuterated alcohols) .
Activité Biologique
Pyrimidine-5-carbaldehyde is a significant compound in medicinal chemistry, particularly due to its diverse biological activities. This article explores the biological properties of this compound, focusing on its synthesis, structure-activity relationships (SAR), and its potential applications in pharmacology.
Synthesis and Structural Characteristics
This compound can be synthesized through various methods, including the condensation of pyrimidine derivatives with aldehydes. The structural features of this compound, particularly the presence of the aldehyde functional group, enhance its reactivity and ability to form Schiff bases, which are crucial for its biological activity.
Biological Activity Overview
Pyrimidine derivatives have been extensively studied for their antibacterial, anticancer, and anti-inflammatory properties. This compound and its derivatives have shown promising results in various biological assays.
Antibacterial Activity
Research indicates that pyrimidine derivatives exhibit significant antibacterial activity against various pathogens. For instance, a study highlighted that certain pyrimidine Schiff bases demonstrated selective antibacterial effects against Enterococcus faecalis, a common nosocomial pathogen. The minimum inhibitory concentration (MIC) for some derivatives was reported as low as 16 µg/mL, indicating potent antibacterial properties .
Anticancer Activity
This compound has been linked to anticancer activity, particularly against gastric adenocarcinoma cell lines. Compounds derived from this compound have shown IC50 values around 53.02 µM for AGS cells, suggesting effective cytotoxicity against cancer cells while maintaining low toxicity towards normal cells .
The biological mechanisms underlying the activity of this compound derivatives include:
- Apoptosis Induction : Some studies suggest that these compounds can induce apoptosis in cancer cells by triggering pathways that lead to programmed cell death .
- Reactive Oxygen Species (ROS) Production : Antibacterial activity may be linked to the generation of ROS, which can damage bacterial cells and inhibit their growth .
- Cell Cycle Arrest : Certain derivatives have been shown to cause cell cycle arrest in the G2/M phase, preventing cancer cells from proliferating .
Case Study 1: Anticancer Activity Against Gastric Cancer
A recent study synthesized a new Schiff base from this compound and evaluated its anticancer properties. The compound exhibited significant cytotoxicity against AGS gastric adenocarcinoma cells with an IC50 value of 32.21 µM. Flow cytometry analysis indicated that the compound induced necrosis rather than apoptosis in these cells .
Case Study 2: Antibacterial Efficacy
Another investigation focused on the antibacterial efficacy of a series of pyrimidine derivatives against E. faecalis. The study revealed that modifications at the 4-position of the pyrimidine ring significantly enhanced antibacterial activity, with some compounds showing synergistic effects when combined with traditional antibiotics like amoxicillin .
Table 1: Biological Activities of this compound Derivatives
Compound Name | Activity Type | Target Organism/Cell Line | MIC/IC50 Value |
---|---|---|---|
Compound III | Antibacterial | E. faecalis | MIC = 16 µg/mL |
Compound IV | Anticancer | AGS (gastric adenocarcinoma) | IC50 = 53.02 µM |
Compound V | Anticancer | A172 (glioblastoma) | IC50 = 63.385 µM |
Q & A
Basic Research Questions
Q. What are the primary synthetic routes for preparing pyrimidine-5-carbaldehyde, and how can reaction conditions be optimized for yield and purity?
this compound is commonly synthesized via formylation of bromo- or iodo-substituted pyrimidines using reagents like DMF/POCl₃ or via coupling reactions with tert-butyldimethylsilylacetylene followed by oxidation . Optimization involves solvent selection (e.g., toluene for reflux conditions), acid catalysts (e.g., p-toluenesulfonic acid), and purification via reduced-pressure distillation or recrystallization. Yield improvements are achieved by controlling stoichiometry and reaction time, as demonstrated in the synthesis of 3-methyl-N-(pyrimidin-5-ylmethyl)pyridin-2-amine .
Q. How can this compound be functionalized to synthesize heterocyclic compounds, and what analytical methods validate these products?
The aldehyde group in this compound reacts with amines, hydrazines, or enolates to form fused heterocycles like pyridopyrimidines or pteridine analogs . For example, condensation with 4-aminopyrimidines under acidic conditions generates di- and tetra-hydropyrimido[4,5-d]pyrimidines. Product validation employs NMR, HPLC, and X-ray crystallography, with chiral columns used to resolve enantiomers in asymmetric reactions .
Advanced Research Questions
Q. What factors govern enantioselectivity in the addition of diisopropylzinc (i-Pr₂Zn) to this compound, and how do chiral initiators influence stereochemical outcomes?
Enantioselectivity depends on the chiral environment created by catalysts or initiators. For instance:
- (R)- or (S)-α-methyl-d₃-alanine induces up to 99% enantiomeric excess (ee) in the resulting pyrimidyl alkanol .
- Chiral mesoporous silica acts as a heterogeneous catalyst, achieving high ee via surface-specific interactions .
- Achiral amines (e.g., N,N’-diethylpiperazine) yield stochastic enantiomer distributions, suggesting spontaneous symmetry breaking under non-directed conditions . Mechanistic studies highlight the role of prochirality orientation in the aldehyde substrate and autocatalytic amplification .
Q. How do heterogeneous reaction conditions (e.g., solid-vapor phase) alter the stereochemical outcomes of this compound reactions compared to liquid-phase systems?
In solid-vapor phase reactions, this compound crystals exhibit random Re/Si face orientations, leading to near-stochastic enantiomer distributions (e.g., S:R ≈ 59:56) due to spatially confined reaction initiation points . This contrasts with liquid-phase systems, where chiral initiators or solvents enforce directional selectivity . Phase-dependent differences in surface energy and molecular mobility are critical for designing enantioselective heterogeneous catalysts.
Q. What methodologies reconcile contradictions in enantioselectivity data between chiral initiator-driven and achiral amine-promoted reactions?
Contradictions arise from competing pathways:
- Chiral initiators (e.g., amino acids) template asymmetric induction via hydrogen bonding or steric effects, overriding stochastic effects .
- Achiral systems rely on crystal packing or statistical fluctuations, producing racemic or near-racemic mixtures . Controlled experiments comparing ee under identical conditions (temperature, solvent, catalyst loading) are essential to isolate variables. Replication studies using single-crystal X-ray analysis of aldehyde substrates can clarify prochirality effects .
Q. How does asymmetric autocatalysis amplify enantiomeric excess in pyrimidyl alkanol synthesis, and what are its limitations?
Asymmetric autocatalysis involves self-replication of chiral alkanol products, which act as catalysts for their own formation. For example:
- Cinnabar with right-handed helicity or chiral NiSO₄·6H₂O crystals initiate autocatalytic cycles, amplifying ee from <1% to >99% . Limitations include sensitivity to competing pathways (e.g., racemization) and dependency on precise stoichiometry. Methodological refinements, such as slow reagent addition and inert-atmosphere protocols, mitigate side reactions .
Q. What strategies optimize the use of this compound in detecting chiral initiators or quantifying enantioselective amplification?
Key strategies include:
- Coupling with HPLC or CD spectroscopy to monitor ee in real-time .
- Varying initiator chirality (e.g., L/D-penicillamine) to correlate configuration with product handedness .
- Temperature modulation (e.g., 0°C for (S)-1-phenethyl alcohol-initiated reactions) to enhance stereocontrol .
Q. Methodological Considerations
Q. How do researchers design controlled experiments to isolate the effects of prochirality in this compound reactions?
- Single-crystal X-ray analysis determines the substrate’s spatial orientation pre-reaction .
- Isotopic labeling (e.g., deuterated solvents) tracks kinetic isotope effects on enantioselectivity .
- Surface-modified catalysts (e.g., silica with chiral ligands) decouple bulk vs. surface contributions .
Q. What are common pitfalls in characterizing this compound derivatives, and how are they addressed?
- Racemization during purification : Minimized via low-temperature chromatography or rapid crystallization .
- Ambiguous NMR assignments : Resolved using 2D-COSY or NOESY to confirm regiochemistry .
- Artifacts in ee measurements : Controlled by replicate HPLC runs with chiral stationary phases .
Propriétés
IUPAC Name |
pyrimidine-5-carbaldehyde | |
---|---|---|
Source | PubChem | |
URL | https://pubchem.ncbi.nlm.nih.gov | |
Description | Data deposited in or computed by PubChem | |
InChI |
InChI=1S/C5H4N2O/c8-3-5-1-6-4-7-2-5/h1-4H | |
Source | PubChem | |
URL | https://pubchem.ncbi.nlm.nih.gov | |
Description | Data deposited in or computed by PubChem | |
InChI Key |
FREJAOSUHFGDBW-UHFFFAOYSA-N | |
Source | PubChem | |
URL | https://pubchem.ncbi.nlm.nih.gov | |
Description | Data deposited in or computed by PubChem | |
Canonical SMILES |
C1=C(C=NC=N1)C=O | |
Source | PubChem | |
URL | https://pubchem.ncbi.nlm.nih.gov | |
Description | Data deposited in or computed by PubChem | |
Molecular Formula |
C5H4N2O | |
Source | PubChem | |
URL | https://pubchem.ncbi.nlm.nih.gov | |
Description | Data deposited in or computed by PubChem | |
DSSTOX Substance ID |
DTXSID10375493 | |
Record name | Pyrimidine-5-carbaldehyde | |
Source | EPA DSSTox | |
URL | https://comptox.epa.gov/dashboard/DTXSID10375493 | |
Description | DSSTox provides a high quality public chemistry resource for supporting improved predictive toxicology. | |
Molecular Weight |
108.10 g/mol | |
Source | PubChem | |
URL | https://pubchem.ncbi.nlm.nih.gov | |
Description | Data deposited in or computed by PubChem | |
CAS No. |
10070-92-5 | |
Record name | Pyrimidine-5-carbaldehyde | |
Source | EPA DSSTox | |
URL | https://comptox.epa.gov/dashboard/DTXSID10375493 | |
Description | DSSTox provides a high quality public chemistry resource for supporting improved predictive toxicology. | |
Record name | Pyrimidine-5-carboxaldehyde | |
Source | European Chemicals Agency (ECHA) | |
URL | https://echa.europa.eu/information-on-chemicals | |
Description | The European Chemicals Agency (ECHA) is an agency of the European Union which is the driving force among regulatory authorities in implementing the EU's groundbreaking chemicals legislation for the benefit of human health and the environment as well as for innovation and competitiveness. | |
Explanation | Use of the information, documents and data from the ECHA website is subject to the terms and conditions of this Legal Notice, and subject to other binding limitations provided for under applicable law, the information, documents and data made available on the ECHA website may be reproduced, distributed and/or used, totally or in part, for non-commercial purposes provided that ECHA is acknowledged as the source: "Source: European Chemicals Agency, http://echa.europa.eu/". Such acknowledgement must be included in each copy of the material. ECHA permits and encourages organisations and individuals to create links to the ECHA website under the following cumulative conditions: Links can only be made to webpages that provide a link to the Legal Notice page. | |
Avertissement et informations sur les produits de recherche in vitro
Veuillez noter que tous les articles et informations sur les produits présentés sur BenchChem sont destinés uniquement à des fins informatives. Les produits disponibles à l'achat sur BenchChem sont spécifiquement conçus pour des études in vitro, qui sont réalisées en dehors des organismes vivants. Les études in vitro, dérivées du terme latin "in verre", impliquent des expériences réalisées dans des environnements de laboratoire contrôlés à l'aide de cellules ou de tissus. Il est important de noter que ces produits ne sont pas classés comme médicaments et n'ont pas reçu l'approbation de la FDA pour la prévention, le traitement ou la guérison de toute condition médicale, affection ou maladie. Nous devons souligner que toute forme d'introduction corporelle de ces produits chez les humains ou les animaux est strictement interdite par la loi. Il est essentiel de respecter ces directives pour assurer la conformité aux normes légales et éthiques en matière de recherche et d'expérimentation.