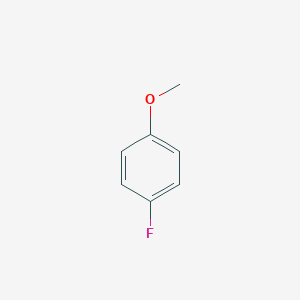
4-Fluoroanisole
Vue d'ensemble
Description
Applications De Recherche Scientifique
4-Fluoroanisole is widely used in scientific research due to its unique properties:
Biology: Its derivatives are used in the study of biological systems and the development of bioactive compounds.
Industry: It is used in the production of liquid crystal materials and other advanced materials.
Mécanisme D'action
Target of Action
4-Fluoroanisole is a chemical compound with the molecular formula FC6H4OCH3 . It’s important to note that the targets can vary depending on the biological system or organism in which the compound is introduced.
Biochemical Pathways
This compound has been mentioned in the context of the Suzuki–Miyaura cross-coupling reaction , a widely applied transition metal catalysed carbon–carbon bond forming reaction.
Méthodes De Préparation
Synthetic Routes and Reaction Conditions: One common synthetic route for 4-Fluoroanisole involves the reaction of 4-fluorophenol with dimethyl sulfate or methyl iodide through an O-alkylation process . Another method includes the reaction of bromofluorobenzene with sodium methoxide in the presence of a solvent like dimethylformamide (DMF) and a catalyst . The reaction mixture is heated and stirred for 10 to 15 hours, followed by distillation to obtain the final product .
Industrial Production Methods: Industrial production of this compound often involves the use of highly efficient catalytic processes to ensure high yield and purity. The use of advanced distillation techniques helps in the separation and purification of the compound .
Analyse Des Réactions Chimiques
Types of Reactions: 4-Fluoroanisole undergoes various types of chemical reactions, including:
Substitution Reactions: It can participate in nucleophilic aromatic substitution reactions due to the presence of the fluorine atom.
Oxidation and Reduction Reactions: The methoxy group can be oxidized or reduced under specific conditions.
Coupling Reactions: It is commonly used in Suzuki–Miyaura coupling reactions to form carbon-carbon bonds.
Common Reagents and Conditions:
Nucleophilic Substitution: Sodium methoxide, DMF as solvent.
Oxidation: Potassium permanganate or other strong oxidizing agents.
Reduction: Lithium aluminum hydride or other reducing agents.
Coupling Reactions: Palladium catalysts, boron reagents.
Major Products: The major products formed from these reactions depend on the specific conditions and reagents used. For example, in Suzuki–Miyaura coupling, the product is typically a biaryl compound .
Comparaison Avec Des Composés Similaires
4-Fluoroanisole can be compared with other fluorinated aromatic ethers such as:
- 3-Fluoroanisole
- 2-Bromo-4-fluoroanisole
- 4-Fluorophenylacetonitrile
Uniqueness: this compound is unique due to its specific substitution pattern, which imparts distinct reactivity and properties. The para-fluorine and methoxy groups make it particularly useful in various synthetic applications, providing a balance of electronic effects that enhance its utility in chemical reactions .
Activité Biologique
4-Fluoroanisole, a fluorinated derivative of anisole, has garnered attention in various fields of biological research due to its unique structural and physicochemical properties. This article explores the biological activity of this compound, focusing on its pharmacological potential, mechanisms of action, and comparative studies with related compounds.
Chemical Structure and Properties
This compound (CHFO) is characterized by a methoxy group (-OCH) and a fluorine atom attached to a benzene ring. The presence of fluorine significantly alters the compound's electronic properties, influencing its biological interactions.
Cholinesterase Inhibition
Recent studies have highlighted the potential of this compound derivatives as cholinesterase inhibitors. A study synthesized several compounds based on 4-fluorobenzoic acid and tetrahydroacridine, evaluating their inhibitory effects on acetylcholinesterase (AChE) and butyrylcholinesterase (BChE). The most promising derivatives showed IC values comparable to tacrine, a known AChE inhibitor, suggesting that this compound derivatives could serve as lead compounds in Alzheimer's disease treatment .
Compound | IC (µM) | Selectivity (AChE/BChE) |
---|---|---|
4a | 12.5 | High |
4d | 10.2 | Highest |
Tacrine | 11.0 | Reference |
Antioxidant Activity
The antioxidant properties of this compound have been explored through various assays. A notable study employed fluorescence techniques to evaluate the reactivity of this compound with lipid peroxyl radicals, demonstrating its potential to inhibit oxidative damage in cellular models . This property is crucial for developing therapeutic agents aimed at conditions associated with oxidative stress.
Fluorination Effects on Drug Design
The introduction of fluorine into drug molecules like this compound can significantly impact their pharmacokinetic profiles. Analysis from Pfizer's corporate database indicated that fluorinated anisoles exhibit altered conformational preferences and enhanced lipophilicity compared to their non-fluorinated counterparts . For instance, the log D values increased substantially with fluorination, although this sometimes resulted in reduced passive permeability.
Property | Anisole (PhOCH) | This compound (PhOCF) |
---|---|---|
Log D | 1.5 | 2.5 |
Passive Permeability | High | Moderate |
Metabolic Stability | Moderate | Low |
Agricultural Applications
Research has also indicated the agricultural potential of compounds derived from this compound. A study reported potent antifungal activities against various plant pathogens, suggesting that derivatives could be developed as effective agricultural fungicides . The compound demonstrated significant herbicidal activity against both dicotyledonous and monocotyledonous plants.
Propriétés
IUPAC Name |
1-fluoro-4-methoxybenzene | |
---|---|---|
Source | PubChem | |
URL | https://pubchem.ncbi.nlm.nih.gov | |
Description | Data deposited in or computed by PubChem | |
InChI |
InChI=1S/C7H7FO/c1-9-7-4-2-6(8)3-5-7/h2-5H,1H3 | |
Source | PubChem | |
URL | https://pubchem.ncbi.nlm.nih.gov | |
Description | Data deposited in or computed by PubChem | |
InChI Key |
VIPWUFMFHBIKQI-UHFFFAOYSA-N | |
Source | PubChem | |
URL | https://pubchem.ncbi.nlm.nih.gov | |
Description | Data deposited in or computed by PubChem | |
Canonical SMILES |
COC1=CC=C(C=C1)F | |
Source | PubChem | |
URL | https://pubchem.ncbi.nlm.nih.gov | |
Description | Data deposited in or computed by PubChem | |
Molecular Formula |
C7H7FO | |
Source | PubChem | |
URL | https://pubchem.ncbi.nlm.nih.gov | |
Description | Data deposited in or computed by PubChem | |
DSSTOX Substance ID |
DTXSID3060033 | |
Record name | p-Fluoroanisole | |
Source | EPA DSSTox | |
URL | https://comptox.epa.gov/dashboard/DTXSID3060033 | |
Description | DSSTox provides a high quality public chemistry resource for supporting improved predictive toxicology. | |
Molecular Weight |
126.13 g/mol | |
Source | PubChem | |
URL | https://pubchem.ncbi.nlm.nih.gov | |
Description | Data deposited in or computed by PubChem | |
CAS No. |
459-60-9 | |
Record name | 4-Fluoroanisole | |
Source | CAS Common Chemistry | |
URL | https://commonchemistry.cas.org/detail?cas_rn=459-60-9 | |
Description | CAS Common Chemistry is an open community resource for accessing chemical information. Nearly 500,000 chemical substances from CAS REGISTRY cover areas of community interest, including common and frequently regulated chemicals, and those relevant to high school and undergraduate chemistry classes. This chemical information, curated by our expert scientists, is provided in alignment with our mission as a division of the American Chemical Society. | |
Explanation | The data from CAS Common Chemistry is provided under a CC-BY-NC 4.0 license, unless otherwise stated. | |
Record name | Benzene, 1-fluoro-4-methoxy- | |
Source | ChemIDplus | |
URL | https://pubchem.ncbi.nlm.nih.gov/substance/?source=chemidplus&sourceid=0000459609 | |
Description | ChemIDplus is a free, web search system that provides access to the structure and nomenclature authority files used for the identification of chemical substances cited in National Library of Medicine (NLM) databases, including the TOXNET system. | |
Record name | 4-Fluoroanisole | |
Source | DTP/NCI | |
URL | https://dtp.cancer.gov/dtpstandard/servlet/dwindex?searchtype=NSC&outputformat=html&searchlist=4672 | |
Description | The NCI Development Therapeutics Program (DTP) provides services and resources to the academic and private-sector research communities worldwide to facilitate the discovery and development of new cancer therapeutic agents. | |
Explanation | Unless otherwise indicated, all text within NCI products is free of copyright and may be reused without our permission. Credit the National Cancer Institute as the source. | |
Record name | Benzene, 1-fluoro-4-methoxy- | |
Source | EPA Chemicals under the TSCA | |
URL | https://www.epa.gov/chemicals-under-tsca | |
Description | EPA Chemicals under the Toxic Substances Control Act (TSCA) collection contains information on chemicals and their regulations under TSCA, including non-confidential content from the TSCA Chemical Substance Inventory and Chemical Data Reporting. | |
Record name | p-Fluoroanisole | |
Source | EPA DSSTox | |
URL | https://comptox.epa.gov/dashboard/DTXSID3060033 | |
Description | DSSTox provides a high quality public chemistry resource for supporting improved predictive toxicology. | |
Record name | 4-fluoroanisole | |
Source | European Chemicals Agency (ECHA) | |
URL | https://echa.europa.eu/substance-information/-/substanceinfo/100.006.633 | |
Description | The European Chemicals Agency (ECHA) is an agency of the European Union which is the driving force among regulatory authorities in implementing the EU's groundbreaking chemicals legislation for the benefit of human health and the environment as well as for innovation and competitiveness. | |
Explanation | Use of the information, documents and data from the ECHA website is subject to the terms and conditions of this Legal Notice, and subject to other binding limitations provided for under applicable law, the information, documents and data made available on the ECHA website may be reproduced, distributed and/or used, totally or in part, for non-commercial purposes provided that ECHA is acknowledged as the source: "Source: European Chemicals Agency, http://echa.europa.eu/". Such acknowledgement must be included in each copy of the material. ECHA permits and encourages organisations and individuals to create links to the ECHA website under the following cumulative conditions: Links can only be made to webpages that provide a link to the Legal Notice page. | |
Record name | 4-Fluoroanisole | |
Source | FDA Global Substance Registration System (GSRS) | |
URL | https://gsrs.ncats.nih.gov/ginas/app/beta/substances/F6A62M57LM | |
Description | The FDA Global Substance Registration System (GSRS) enables the efficient and accurate exchange of information on what substances are in regulated products. Instead of relying on names, which vary across regulatory domains, countries, and regions, the GSRS knowledge base makes it possible for substances to be defined by standardized, scientific descriptions. | |
Explanation | Unless otherwise noted, the contents of the FDA website (www.fda.gov), both text and graphics, are not copyrighted. They are in the public domain and may be republished, reprinted and otherwise used freely by anyone without the need to obtain permission from FDA. Credit to the U.S. Food and Drug Administration as the source is appreciated but not required. | |
Retrosynthesis Analysis
AI-Powered Synthesis Planning: Our tool employs the Template_relevance Pistachio, Template_relevance Bkms_metabolic, Template_relevance Pistachio_ringbreaker, Template_relevance Reaxys, Template_relevance Reaxys_biocatalysis model, leveraging a vast database of chemical reactions to predict feasible synthetic routes.
One-Step Synthesis Focus: Specifically designed for one-step synthesis, it provides concise and direct routes for your target compounds, streamlining the synthesis process.
Accurate Predictions: Utilizing the extensive PISTACHIO, BKMS_METABOLIC, PISTACHIO_RINGBREAKER, REAXYS, REAXYS_BIOCATALYSIS database, our tool offers high-accuracy predictions, reflecting the latest in chemical research and data.
Strategy Settings
Precursor scoring | Relevance Heuristic |
---|---|
Min. plausibility | 0.01 |
Model | Template_relevance |
Template Set | Pistachio/Bkms_metabolic/Pistachio_ringbreaker/Reaxys/Reaxys_biocatalysis |
Top-N result to add to graph | 6 |
Feasible Synthetic Routes
A: 4-Fluoroanisole forms inclusion complexes with α- and β-cyclodextrins, effectively shielding it from nucleophilic attack during photochemical reactions like photocyanation and photohydroxylation. [] This complexation significantly suppresses the photoreactivity of this compound. [] Interestingly, γ-cyclodextrin shows a negligible effect on these photosubstitution reactions, and the reasons are still being investigated. []
ANone:
- Spectroscopic Data:
- NMR: Long-range coupling constants over six bonds between 13C nuclei in the methyl group and ring protons or 19F nuclei have been reported. [] These couplings provide valuable insights into the conformational properties of this compound. []
- IR and Raman: Detailed vibrational analysis of this compound has been performed using infrared and Raman spectroscopy, supported by normal coordinate analysis. [] This provides a comprehensive understanding of the molecule's vibrational modes. []
- 19F NMR: The 19F NMR chemical shifts of this compound in various solvents are used to quantify solvent hydrogen-bond basicity. [] This method, termed the solvatomagnetic comparison method, helps understand the interaction of this compound with different solvents. []
A: Solvent mixtures, such as water and tert-butyl alcohol, exhibit unique effects on the aromatic nucleophilic photosubstitutions of this compound. [] The specific solvent composition significantly influences the reaction pathway and product distribution, highlighting the importance of solvent selection in these reactions. []
ANone: Yes, this compound serves as a versatile starting material in organic synthesis. For instance:
- Fidarestat Synthesis: It's a key precursor in the multi-step synthesis of Fidarestat, a drug candidate for treating diabetic complications. []
- Alkyl-Lithium Reactions: Reactions with alkyl-lithium reagents, followed by carbonation, yield 6-alkyl-2-methoxybenzoic acids. [] These reactions involve the formation of methoxybenzynes as intermediates. []
- Aryl Grignard Reagent Formation: this compound can be converted to its corresponding aryl Grignard reagent, which then facilitates the synthesis of this compound itself, showcasing a unique synthetic approach. []
ANone: Yes, computational chemistry plays a crucial role in understanding this compound:
- Proton Affinity Calculations: Ab initio calculations, such as G3, G3(MP2), and MP2 methods, have been employed to determine the proton affinities of this compound and its derivatives. [] These calculations provide insights into the basicity of different sites within the molecule. []
- Solvent Parameter Databases: this compound acts as a probe molecule in establishing databases of solvent parameters, particularly for quantifying hydrogen-bond acceptor (β1) properties. [] This data aids in understanding and predicting solvent effects on various physicochemical properties. []
- Mechanistic Studies of C-H Activation: Computational studies using anisole, structurally similar to this compound, have helped elucidate the mechanism of triple C-H bond activation by iridium complexes. [] This provides valuable insights into the reactivity of these compounds in catalytic systems. []
ANone: Structural modifications significantly impact the reactivity of this compound:
- Nitration Reactions: Studies on the nitration of 4-substituted anisoles, including those with chlorine and methyl groups, reveal the influence of substituents on the regioselectivity and reaction rates. [] The formation and rearrangement of 4-nitro-4-substituted-cyclohexa-2,5-dienone intermediates are key aspects of these reactions. []
- Photochemical Reactions: Comparing the photochemical behavior of this compound with its 2-fluoroanisole counterpart reveals the impact of fluorine position on photohydroxylation and photocyanation. [] The relative positions of the fluorine and methoxy groups influence the reaction pathways and product distributions. []
Avertissement et informations sur les produits de recherche in vitro
Veuillez noter que tous les articles et informations sur les produits présentés sur BenchChem sont destinés uniquement à des fins informatives. Les produits disponibles à l'achat sur BenchChem sont spécifiquement conçus pour des études in vitro, qui sont réalisées en dehors des organismes vivants. Les études in vitro, dérivées du terme latin "in verre", impliquent des expériences réalisées dans des environnements de laboratoire contrôlés à l'aide de cellules ou de tissus. Il est important de noter que ces produits ne sont pas classés comme médicaments et n'ont pas reçu l'approbation de la FDA pour la prévention, le traitement ou la guérison de toute condition médicale, affection ou maladie. Nous devons souligner que toute forme d'introduction corporelle de ces produits chez les humains ou les animaux est strictement interdite par la loi. Il est essentiel de respecter ces directives pour assurer la conformité aux normes légales et éthiques en matière de recherche et d'expérimentation.