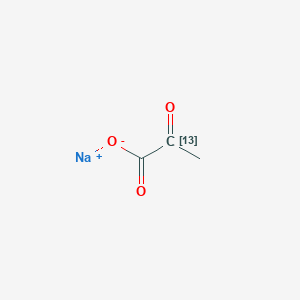
Pyruvate de sodium-2-13C
Vue d'ensemble
Description
Sodium pyruvate-2-13C is a stable isotope-labeled compound where the second carbon atom in the pyruvate molecule is replaced with the carbon-13 isotope. This compound is widely used in biochemical and metabolic research due to its ability to trace metabolic pathways and study enzyme kinetics. The molecular formula of sodium pyruvate-2-13C is CH3COCO2Na, and it has a molecular weight of 111.04 g/mol .
Applications De Recherche Scientifique
Metabolic Flux Analysis
Sodium pyruvate-2-13C is extensively utilized in metabolic flux analysis to study cellular metabolism. By tracking the incorporation of the 13C label into various metabolites, researchers can gain insights into metabolic pathways and energy production.
Key Findings:
- Cellular Respiration : It provides a means to investigate how cells utilize pyruvate in the citric acid cycle, which is crucial for ATP production.
- Cancer Research : The Warburg effect, where cancer cells preferentially use glycolysis followed by lactic acid fermentation, can be studied using this compound to observe altered metabolic pathways in tumor cells .
NMR Spectroscopy
The compound is employed in NMR spectroscopy to investigate molecular structures and dynamics. Its isotopic labeling enhances the sensitivity and resolution of NMR experiments.
Applications:
- Structural Biology : Used to determine the structure of biomolecules and their interactions.
- Dynamics Studies : Helps in understanding conformational changes in proteins and other macromolecules.
Medical Imaging
Sodium pyruvate-2-13C has significant potential in medical diagnostics, particularly through hyperpolarized magnetic resonance imaging (MRI). This technique allows for real-time monitoring of metabolic processes in vivo.
Clinical Applications:
- Cancer Diagnosis : Hyperpolarized [1-13C]pyruvate has been investigated for its ability to differentiate between healthy and cancerous tissues based on metabolic activity .
- Cardiovascular Studies : It aids in assessing cardiac metabolism and identifying conditions such as cardiomyopathy through altered metabolic patterns observed via imaging techniques .
Case Study 1: Hyperpolarized [1-13C]Pyruvate in Cancer Imaging
A study demonstrated the effectiveness of hyperpolarized [1-13C]pyruvate in detecting prostate cancer. The research involved administering the compound to animal models and utilizing MRI to visualize metabolic changes. Results indicated a significant increase in lactate production in cancerous tissues compared to normal tissues, validating its potential as a diagnostic tool .
Case Study 2: Metabolic Imaging of Cardiac Function
Another investigation utilized hyperpolarized [1-13C]pyruvate to study cardiac metabolism in mice models with dilated cardiomyopathy. The study revealed that the rate of conversion from pyruvate to lactate was significantly altered, providing insights into the metabolic dysfunction associated with heart disease .
Data Tables
Application Area | Specific Use Cases | Key Benefits |
---|---|---|
Metabolic Flux Analysis | Studying glycolysis and TCA cycle dynamics | Insights into energy metabolism |
NMR Spectroscopy | Analyzing biomolecular structures | Enhanced sensitivity and resolution |
Medical Imaging | Diagnosing cancer and cardiovascular conditions | Real-time monitoring of metabolism |
Mécanisme D'action
Target of Action
Sodium pyruvate-2-13C is an isotopic analogue of sodium pyruvate . The primary target of sodium pyruvate is the pyruvate dehydrogenase complex (PDC), a key enzyme in the metabolic pathway that converts carbohydrates into energy .
Mode of Action
Sodium pyruvate-2-13C interacts with its target, the PDC, by providing an alternative source of pyruvate, which is a critical component in the citric acid cycle (also known as the Krebs cycle or TCA cycle). This cycle is a series of chemical reactions used by all aerobic organisms to generate energy .
Biochemical Pathways
The primary biochemical pathway affected by sodium pyruvate-2-13C is the citric acid cycle. Pyruvate, the product of glycolysis, enters the mitochondria where it is converted to acetyl CoA by the PDC. Acetyl CoA then enters the citric acid cycle, resulting in the production of ATP, the main energy currency of the cell .
Pharmacokinetics
It is known that pyruvate, the non-isotopic form of the compound, is rapidly absorbed and distributed throughout the body
Result of Action
The primary result of sodium pyruvate-2-13C action is the enhancement of cellular energy production. By providing an additional source of pyruvate, sodium pyruvate-2-13C can increase the efficiency of the citric acid cycle, leading to increased ATP production .
Action Environment
The action of sodium pyruvate-2-13C is influenced by various environmental factors. For instance, the presence of other substrates and cofactors necessary for the citric acid cycle can affect the efficacy of sodium pyruvate-2-13C. Additionally, the pH and temperature of the cellular environment can impact the stability and activity of the compound .
Analyse Biochimique
Biochemical Properties
Sodium Pyruvate-2-13C interacts with several enzymes, proteins, and other biomolecules. It is a key molecule in energy production and functions as an antioxidant . It is incorporated into cells via monocarboxylate transporters and predominantly functions as an antioxidant .
Cellular Effects
Sodium Pyruvate-2-13C has significant effects on various types of cells and cellular processes. It influences cell function, including impacts on cell signaling pathways, gene expression, and cellular metabolism . In adipocytes, sodium pyruvate promotes increased uptake of insulin-mediated glucose .
Molecular Mechanism
Sodium Pyruvate-2-13C exerts its effects at the molecular level through various mechanisms. One way in which sodium pyruvate provides energy to cells is through its conversion to acetyl-CoA, which then enters the TCA cycle, producing energy and linking to other energy-producing processes .
Temporal Effects in Laboratory Settings
The effects of Sodium Pyruvate-2-13C change over time in laboratory settings. It has been observed that an increase in maximal mitochondrial respiration occurs in cells upon PDK inhibition by Sodium Pyruvate-2-13C, in parallel with less proliferative capacity .
Dosage Effects in Animal Models
The effects of Sodium Pyruvate-2-13C vary with different dosages in animal models. For instance, intravenous administrations of bolus (500 mg/kg) and continuous infusion (1000 mg/kg/h) of Sodium Pyruvate-2-13C successfully increased oxygen metabolism in post 10-min CA rats .
Metabolic Pathways
Sodium Pyruvate-2-13C is involved in several metabolic pathways. It is a key intermediate in the metabolism of each cell, linking the anaerobic and aerobic portions of glucose catabolism . It also plays a central role in the metabolism of the Central Nervous System (CNS), linking the anaerobic and aerobic portions of glucose catabolism .
Transport and Distribution
Sodium Pyruvate-2-13C is transported and distributed within cells and tissues. It is incorporated into cells via monocarboxylate transporters . In the body, sodium pyruvate provides energy to cells through its conversion to acetyl-CoA, which then can enter the TCA cycle .
Subcellular Localization
The subcellular localization of Sodium Pyruvate-2-13C and its effects on activity or function are significant. For instance, ectopically expressed PDK2 and PDK3, which interact with Sodium Pyruvate-2-13C, were mainly localized in mitochondria compartments .
Méthodes De Préparation
Synthetic Routes and Reaction Conditions: Sodium pyruvate-2-13C can be synthesized through the carboxylation of labeled acetaldehyde. The reaction involves the use of carbon-13 labeled carbon dioxide in the presence of a base to form the labeled pyruvate. The reaction conditions typically include a controlled temperature and pressure to ensure the incorporation of the carbon-13 isotope.
Industrial Production Methods: In industrial settings, sodium pyruvate-2-13C is produced using isotopically labeled precursors. The process involves the fermentation of labeled glucose by microorganisms, followed by the extraction and purification of the labeled pyruvate. The final product is then converted to its sodium salt form through neutralization with sodium hydroxide .
Types of Reactions:
Oxidation: Sodium pyruvate-2-13C can undergo oxidation to form carbon dioxide and water. This reaction is catalyzed by pyruvate dehydrogenase in the presence of coenzymes.
Reduction: It can be reduced to lactate by lactate dehydrogenase in anaerobic conditions.
Substitution: Sodium pyruvate-2-13C can participate in nucleophilic substitution reactions, where the carboxylate group can be replaced by other nucleophiles.
Common Reagents and Conditions:
Oxidation: Pyruvate dehydrogenase complex, NAD+, and coenzyme A.
Reduction: Lactate dehydrogenase and NADH.
Substitution: Various nucleophiles such as amines or thiols under basic conditions.
Major Products:
Oxidation: Acetyl-CoA, carbon dioxide, and NADH.
Reduction: Lactate and NAD+.
Substitution: Corresponding substituted pyruvate derivatives.
Comparaison Avec Des Composés Similaires
Sodium pyruvate-1-13C: Labeled at the first carbon atom.
Sodium pyruvate-3-13C: Labeled at the third carbon atom.
Sodium pyruvate-2,3-13C2: Labeled at both the second and third carbon atoms.
Uniqueness: Sodium pyruvate-2-13C is unique due to its specific labeling at the second carbon atom, which allows for detailed studies of metabolic pathways involving this position. This specificity is crucial for understanding the role of the second carbon in enzymatic reactions and metabolic flux .
Activité Biologique
Sodium pyruvate-2-13C is a stable isotope-labeled compound that plays a significant role in metabolic research, particularly in the study of cellular metabolism and energy production. This article explores the biological activity of sodium pyruvate-2-13C, focusing on its metabolic pathways, applications in imaging techniques, and implications for disease characterization.
Overview of Sodium Pyruvate-2-13C
Sodium pyruvate is a key intermediate in several metabolic pathways, including glycolysis and the citric acid cycle. The incorporation of the carbon-13 isotope enhances the ability to trace metabolic processes using magnetic resonance spectroscopy (MRS) and other imaging techniques. The compound is primarily used in studies involving hyperpolarization techniques, which significantly increase the sensitivity of MRS.
Metabolic Pathways
Sodium pyruvate-2-13C is involved in various metabolic pathways:
- Glycolysis : Pyruvate is produced from glucose through glycolysis and can be converted into lactate or acetyl-CoA.
- Citric Acid Cycle : Pyruvate is decarboxylated to acetyl-CoA by pyruvate dehydrogenase (PDH), entering the citric acid cycle for further energy production.
The following table summarizes the key metabolites derived from sodium pyruvate-2-13C:
Metabolite | Pathway | Function |
---|---|---|
Lactate | Anaerobic glycolysis | Energy production under low oxygen conditions |
Acetyl-CoA | Citric acid cycle | Central role in energy metabolism |
Bicarbonate | Mitochondrial metabolism | Indicator of PDH activity |
Glutamate | Amino acid metabolism | Neurotransmitter synthesis |
Hyperpolarization Techniques
The use of hyperpolarized sodium pyruvate-2-13C has revolutionized metabolic imaging. Hyperpolarization increases the signal-to-noise ratio (SNR) in MRS, allowing for real-time observation of metabolic processes. Studies have shown that:
- Increased Conversion Rates : Following administration of dichloroacetate (DCA), a significant increase in bicarbonate production was observed, indicating enhanced PDH activity. Specifically, a study noted an increase of 181% ± 69% in bicarbonate levels after DCA administration .
- Metabolic Imaging : In vivo studies using hyperpolarized [1-13C]pyruvate have successfully mapped tissue-specific localization of metabolites such as lactate and acetyl-CoA, demonstrating the compound's utility in characterizing metabolic states in various tissues, including cancerous tissues .
Case Studies
Several case studies illustrate the biological activity and clinical relevance of sodium pyruvate-2-13C:
- Cancer Metabolism : In a study involving prostate cancer models, hyperpolarized [1-13C]pyruvate was used to detect increased lactate production, a hallmark of the Warburg effect, where cancer cells favor anaerobic metabolism even in the presence of oxygen . This finding underscores the potential for using sodium pyruvate-2-13C as a biomarker for cancer diagnosis.
- Cardiac Function : Research on dilated cardiomyopathy models demonstrated altered pyruvate metabolism, with reduced lactate production observed in affected hearts compared to controls. This suggests that sodium pyruvate-2-13C can serve as an indicator of cardiac health and disease progression .
Research Findings
Recent findings highlight the versatility and importance of sodium pyruvate-2-13C in metabolic research:
- Mitochondrial Function : Studies have shown that sodium pyruvate can be used to probe mitochondrial metabolism effectively. The conversion rates to various metabolites provide insights into mitochondrial health and function .
- Clinical Applications : Ongoing clinical trials are exploring the use of hyperpolarized [1-13C]pyruvate for non-invasive imaging techniques to assess tumor metabolism and response to therapy .
Propriétés
IUPAC Name |
sodium;2-oxo(213C)propanoate | |
---|---|---|
Source | PubChem | |
URL | https://pubchem.ncbi.nlm.nih.gov | |
Description | Data deposited in or computed by PubChem | |
InChI |
InChI=1S/C3H4O3.Na/c1-2(4)3(5)6;/h1H3,(H,5,6);/q;+1/p-1/i2+1; | |
Source | PubChem | |
URL | https://pubchem.ncbi.nlm.nih.gov | |
Description | Data deposited in or computed by PubChem | |
InChI Key |
DAEPDZWVDSPTHF-CGOMOMTCSA-M | |
Source | PubChem | |
URL | https://pubchem.ncbi.nlm.nih.gov | |
Description | Data deposited in or computed by PubChem | |
Canonical SMILES |
CC(=O)C(=O)[O-].[Na+] | |
Source | PubChem | |
URL | https://pubchem.ncbi.nlm.nih.gov | |
Description | Data deposited in or computed by PubChem | |
Isomeric SMILES |
C[13C](=O)C(=O)[O-].[Na+] | |
Source | PubChem | |
URL | https://pubchem.ncbi.nlm.nih.gov | |
Description | Data deposited in or computed by PubChem | |
Molecular Formula |
C3H3NaO3 | |
Source | PubChem | |
URL | https://pubchem.ncbi.nlm.nih.gov | |
Description | Data deposited in or computed by PubChem | |
DSSTOX Substance ID |
DTXSID60583980 | |
Record name | Sodium 2-oxo(2-~13~C)propanoate | |
Source | EPA DSSTox | |
URL | https://comptox.epa.gov/dashboard/DTXSID60583980 | |
Description | DSSTox provides a high quality public chemistry resource for supporting improved predictive toxicology. | |
Molecular Weight |
111.04 g/mol | |
Source | PubChem | |
URL | https://pubchem.ncbi.nlm.nih.gov | |
Description | Data deposited in or computed by PubChem | |
CAS No. |
87976-70-3 | |
Record name | Sodium 2-oxo(2-~13~C)propanoate | |
Source | EPA DSSTox | |
URL | https://comptox.epa.gov/dashboard/DTXSID60583980 | |
Description | DSSTox provides a high quality public chemistry resource for supporting improved predictive toxicology. | |
Retrosynthesis Analysis
AI-Powered Synthesis Planning: Our tool employs the Template_relevance Pistachio, Template_relevance Bkms_metabolic, Template_relevance Pistachio_ringbreaker, Template_relevance Reaxys, Template_relevance Reaxys_biocatalysis model, leveraging a vast database of chemical reactions to predict feasible synthetic routes.
One-Step Synthesis Focus: Specifically designed for one-step synthesis, it provides concise and direct routes for your target compounds, streamlining the synthesis process.
Accurate Predictions: Utilizing the extensive PISTACHIO, BKMS_METABOLIC, PISTACHIO_RINGBREAKER, REAXYS, REAXYS_BIOCATALYSIS database, our tool offers high-accuracy predictions, reflecting the latest in chemical research and data.
Strategy Settings
Precursor scoring | Relevance Heuristic |
---|---|
Min. plausibility | 0.01 |
Model | Template_relevance |
Template Set | Pistachio/Bkms_metabolic/Pistachio_ringbreaker/Reaxys/Reaxys_biocatalysis |
Top-N result to add to graph | 6 |
Feasible Synthetic Routes
Avertissement et informations sur les produits de recherche in vitro
Veuillez noter que tous les articles et informations sur les produits présentés sur BenchChem sont destinés uniquement à des fins informatives. Les produits disponibles à l'achat sur BenchChem sont spécifiquement conçus pour des études in vitro, qui sont réalisées en dehors des organismes vivants. Les études in vitro, dérivées du terme latin "in verre", impliquent des expériences réalisées dans des environnements de laboratoire contrôlés à l'aide de cellules ou de tissus. Il est important de noter que ces produits ne sont pas classés comme médicaments et n'ont pas reçu l'approbation de la FDA pour la prévention, le traitement ou la guérison de toute condition médicale, affection ou maladie. Nous devons souligner que toute forme d'introduction corporelle de ces produits chez les humains ou les animaux est strictement interdite par la loi. Il est essentiel de respecter ces directives pour assurer la conformité aux normes légales et éthiques en matière de recherche et d'expérimentation.