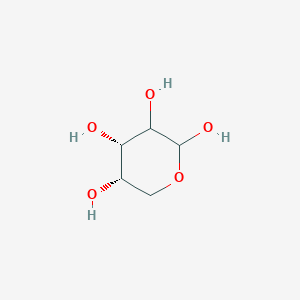
L-Arabinose
Vue d'ensemble
Description
L-Arabinose est un aldopentose naturel, un monosaccharide contenant cinq atomes de carbone. On le trouve principalement dans les plantes, notamment dans le maïs, les patates douces et diverses autres matières végétales. This compound est connu pour sa propriété unique d'avoir environ 50 % de la douceur du saccharose. Contrairement aux autres sucres, this compound n'est pas facilement absorbé par l'intestin grêle, ce qui en fait un édulcorant sans calorie .
Applications De Recherche Scientifique
L-Arabinose has a wide range of applications in scientific research:
Chemistry: Used as a precursor in the synthesis of various compounds.
Medicine: Investigated for its potential in controlling hyperglycemia and improving insulin resistance.
Industry: Used as a low-calorie sweetener and in the production of functional foods.
Mécanisme D'action
Target of Action
Arabinopyranoside primarily targets α-L-arabinofuranosidases (EC3.2.1.55), enzymes that play a crucial role in the degradation of lignocelluloses . These enzymes have received increased attention due to their positive effect on the activity of other enzymes acting on lignocelluloses .
Mode of Action
Arabinopyranoside interacts with its targets through a process of hydrolysis. The α-L-arabinofuranosidases cleave α-L-arabinofuranosidic linkages and act synergistically with other hemicellulases and pectic enzymes for the complete hydrolysis of hemicelluloses and pectins . This interaction results in significant changes in the structure and function of the target enzymes, enhancing their activity and effectiveness .
Biochemical Pathways
Arabinopyranoside affects several biochemical pathways, particularly those involved in the degradation of lignocelluloses. L-arabinosyl residues are widely distributed in these polymers as side chains . The presence of these side chains restricts the enzymatic hydrolysis of hemicelluloses and pectins . Arabinopyranoside, through its action on α-L-arabinofuranosidases, facilitates the hydrolysis of these polymers, thereby affecting the overall biochemical pathway of lignocellulose degradation .
Pharmacokinetics
It’s worth noting that the bioavailability of similar compounds, such as myricetin, is known to be low due to scarce absorption
Result of Action
The action of Arabinopyranoside results in the enhanced degradation of lignocelluloses. This is achieved through the hydrolysis of α-L-arabinofuranosidic linkages, which facilitates the complete hydrolysis of hemicelluloses and pectins . This action has significant molecular and cellular effects, including the release of L-arabinosyl moiety as the main product .
Action Environment
The action, efficacy, and stability of Arabinopyranoside can be influenced by various environmental factors. For instance, the chemical stability of similar compounds, such as myricetin, is known to be pH and temperature-dependent . Depending on the environmental conditions, these compounds can exert both potent antioxidant and pro-oxidant effects
Analyse Biochimique
Biochemical Properties
L-Arabinose plays a significant role in biochemical reactions. The strain Escherichia coli can use this compound as a sole carbon source for growth . The encoded this compound Isomerase can catalyze this compound to L-Ribulose . L-Ribulose is further transformed to L-Ribulose-5-phosphate by L-Ribulokinase .
Cellular Effects
This compound can inhibit sucrose absorption, improve insulin resistance, reduce serum triglyceride, reduce fat production, and improve the intestinal environment . These effects influence cell function, including impact on cell signaling pathways, gene expression, and cellular metabolism .
Molecular Mechanism
The molecular mechanism of this compound involves its conversion to L-Ribulose by the enzyme this compound Isomerase . This enzyme-catalyzed reaction is a key step in the metabolic pathway that allows certain bacteria to use this compound as a sole carbon source .
Temporal Effects in Laboratory Settings
It is known that this compound Isomerase, the enzyme that catalyzes the conversion of this compound to L-Ribulose, exhibits the greatest activity at 50°C, pH 7–7.5 .
Metabolic Pathways
This compound is involved in a metabolic pathway where it is converted to L-Ribulose by the enzyme this compound Isomerase . L-Ribulose is then transformed to L-Ribulose-5-phosphate by L-Ribulokinase .
Méthodes De Préparation
Voies de synthèse et conditions de réaction : L-Arabinose peut être synthétisé par diverses méthodes. Une méthode nouvelle consiste en la biopurification de la liqueur mère de xylose à l'aide de souches de levures qui métabolisent d'autres sucres, mais pas this compound. Cette méthode implique une fermentation dans un milieu contenant de la liqueur mère de xylose, en optimisant les conditions telles que le temps de fermentation et la température pour obtenir une this compound de haute pureté .
Méthodes de production industrielle : La production industrielle de this compound implique souvent l'hydrolyse de l'arabinoxylane, un composant de la fibre de maïs, à l'aide d'enzymes telles que la β-xylanase, la β-xylosidase et l'α-L-arabinofuranosidase. L'hydrolysat obtenu est ensuite soumis à une fermentation sélective avec des levures pour éliminer les autres sucres, suivie de procédés de purification comprenant la décoloration et la déionisation . Une autre méthode implique l'hydrolyse de la pectine dans la pulpe de betterave sucrière, suivie d'une série d'étapes de purification pour obtenir de la this compound cristalline .
Analyse Des Réactions Chimiques
Types de réactions : L-Arabinose subit diverses réactions chimiques, notamment l'isomérisation, l'oxydation et la réduction. Une réaction notable est l'isomérisation de this compound en L-Ribulose, D-Galactose et D-Tagatose, catalysée par l'isomérase this compound .
Réactifs et conditions courants :
Isomérisation : Catalysée par l'isomérase this compound dans des conditions spécifiques.
Oxydation : this compound peut être oxydé pour former de l'acide arabinonique à l'aide d'agents oxydants.
Réduction : La réduction de this compound peut produire de l'arabinitol.
Principaux produits :
L-Ribulose : Formé par isomérisation.
Acide arabinonique : Formé par oxydation.
Arabinitol : Formé par réduction.
4. Applications de la recherche scientifique
This compound a une large gamme d'applications dans la recherche scientifique :
Chimie : Utilisé comme précurseur dans la synthèse de divers composés.
Industrie : Utilisé comme édulcorant faible en calories et dans la production d'aliments fonctionnels.
5. Mécanisme d'action
This compound exerce ses effets principalement en inhibant l'enzyme sucrase, qui est responsable de la dégradation du saccharose en glucose et en fructose. En inhibant la sucrase, this compound réduit l'absorption du saccharose dans l'intestin grêle, ce qui entraîne une diminution de la glycémie . De plus, this compound peut améliorer la résistance à l'insuline et réduire les triglycérides sériques, contribuant à ses effets bénéfiques sur la santé métabolique .
Composés similaires :
D-Arabinose : Un autre aldopentose, mais moins courant dans la nature que this compound.
Ribose : Un aldopentose similaire, mais avec des rôles biologiques différents.
Xylose : Un autre sucre à cinq atomes de carbone, couramment trouvé dans l'hémicellulose.
Unicité de this compound : this compound est unique en raison de sa capacité à inhiber la sucrase et de sa faible valeur calorique, ce qui en fait une alternative intéressante aux sucres traditionnels. Sa présence dans diverses matières végétales et son rôle dans la santé métabolique le distinguent encore des autres composés similaires .
Comparaison Avec Des Composés Similaires
D-Arabinose: Another aldopentose, but less common in nature compared to L-Arabinose.
Ribose: A similar aldopentose, but with different biological roles.
Xylose: Another five-carbon sugar, commonly found in hemicellulose.
Uniqueness of this compound: this compound is unique due to its ability to inhibit sucrase and its low caloric value, making it an attractive alternative to traditional sugars. Its presence in various plant materials and its role in metabolic health further distinguish it from other similar compounds .
Propriétés
{ "Design of the Synthesis Pathway": "L-Arabinose can be synthesized from D-galactose through a series of enzymatic reactions.", "Starting Materials": [ "D-galactose", "NADPH", "ATP", "Mg2+", "H2O", "O2", "Glucose-1-phosphate" ], "Reaction": [ "D-galactose is first converted to galactose-1-phosphate by the enzyme galactokinase, using ATP as a phosphate donor.", "Galactose-1-phosphate is then converted to UDP-galactose by the enzyme UDP-galactose-4-epimerase, using NADPH as a cofactor.", "UDP-galactose is then converted to UDP-glucose by the enzyme UDP-glucose-4-epimerase.", "UDP-glucose is then converted to glucose-1-phosphate by the enzyme UDP-glucose pyrophosphorylase, using UTP as a substrate.", "Glucose-1-phosphate is then converted to L-arabinose-5-phosphate by the enzyme L-arabinose-5-phosphate isomerase.", "L-arabinose-5-phosphate is then converted to L-arabinose by the enzyme phosphatase, releasing phosphate as a byproduct." ] } | |
Numéro CAS |
87-72-9 |
Formule moléculaire |
C5H10O5 |
Poids moléculaire |
150.13 g/mol |
Nom IUPAC |
(2S,3S,4S,5S)-oxane-2,3,4,5-tetrol |
InChI |
InChI=1S/C5H10O5/c6-2-1-10-5(9)4(8)3(2)7/h2-9H,1H2/t2-,3-,4-,5-/m0/s1 |
Clé InChI |
SRBFZHDQGSBBOR-FCAWWPLPSA-N |
SMILES isomérique |
C1[C@@H]([C@@H]([C@@H]([C@H](O1)O)O)O)O |
SMILES |
C1C(C(C(C(O1)O)O)O)O |
SMILES canonique |
C1C(C(C(C(O1)O)O)O)O |
melting_point |
158 - 160 °C |
28697-53-2 50986-18-0 87-72-9 |
|
Description physique |
White odorless powder; [Alfa Aesar MSDS] |
Solubilité |
500.0 mg/mL |
Synonymes |
Arabinose L Arabinose L-Arabinose |
Origine du produit |
United States |
Retrosynthesis Analysis
AI-Powered Synthesis Planning: Our tool employs the Template_relevance Pistachio, Template_relevance Bkms_metabolic, Template_relevance Pistachio_ringbreaker, Template_relevance Reaxys, Template_relevance Reaxys_biocatalysis model, leveraging a vast database of chemical reactions to predict feasible synthetic routes.
One-Step Synthesis Focus: Specifically designed for one-step synthesis, it provides concise and direct routes for your target compounds, streamlining the synthesis process.
Accurate Predictions: Utilizing the extensive PISTACHIO, BKMS_METABOLIC, PISTACHIO_RINGBREAKER, REAXYS, REAXYS_BIOCATALYSIS database, our tool offers high-accuracy predictions, reflecting the latest in chemical research and data.
Strategy Settings
Precursor scoring | Relevance Heuristic |
---|---|
Min. plausibility | 0.01 |
Model | Template_relevance |
Template Set | Pistachio/Bkms_metabolic/Pistachio_ringbreaker/Reaxys/Reaxys_biocatalysis |
Top-N result to add to graph | 6 |
Feasible Synthetic Routes
Avertissement et informations sur les produits de recherche in vitro
Veuillez noter que tous les articles et informations sur les produits présentés sur BenchChem sont destinés uniquement à des fins informatives. Les produits disponibles à l'achat sur BenchChem sont spécifiquement conçus pour des études in vitro, qui sont réalisées en dehors des organismes vivants. Les études in vitro, dérivées du terme latin "in verre", impliquent des expériences réalisées dans des environnements de laboratoire contrôlés à l'aide de cellules ou de tissus. Il est important de noter que ces produits ne sont pas classés comme médicaments et n'ont pas reçu l'approbation de la FDA pour la prévention, le traitement ou la guérison de toute condition médicale, affection ou maladie. Nous devons souligner que toute forme d'introduction corporelle de ces produits chez les humains ou les animaux est strictement interdite par la loi. Il est essentiel de respecter ces directives pour assurer la conformité aux normes légales et éthiques en matière de recherche et d'expérimentation.