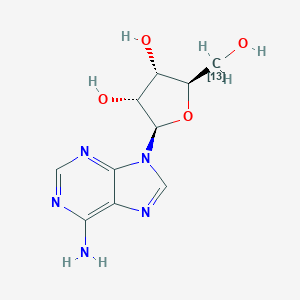
adenosine-5'-13C
Vue d'ensemble
Description
Adenosine-5'-13C (CAS: 54447-57-3) is a stable isotope-labeled derivative of adenosine, where the 13C isotope is incorporated at the 5' carbon position of the ribose moiety. This compound is primarily utilized in nuclear magnetic resonance (NMR) spectroscopy and mass spectrometry-based metabolic tracing studies, enabling researchers to track adenosine incorporation into nucleic acids, coenzymes, and signaling molecules like ATP . Its synthesis involves 13C-enriched precursors, as demonstrated in methods for purine labeling . Unlike non-labeled adenosine, the isotopic label allows precise detection in complex biological matrices, making it indispensable in pharmacokinetic and metabolic flux analyses .
Méthodes De Préparation
Synthetic Routes and Reaction Conditions
The synthesis of adenosine-5'-13C typically involves multi-step organic reactions. The process begins with the preparation of the sugar moiety, followed by the attachment of the purine base. Key steps include:
Glycosylation: The sugar moiety is prepared through glycosylation reactions, where a protected sugar derivative reacts with a purine base.
Deprotection: Protective groups are removed under specific conditions to yield the final nucleoside analog.
Industrial Production Methods
Industrial production of this compound involves large-scale synthesis using optimized reaction conditions to ensure high yield and purity. Techniques such as column chromatography and crystallization are employed to purify the final product.
Analyse Des Réactions Chimiques
Substitution Reactions
Adenosine-5'-13C undergoes regioselective substitutions at the purine base or ribose moiety:
-
6-Chloropurine intermediate : Selective substitution at the C6 position occurs using palladium-catalyzed cross-coupling with alkynes or alkyl groups to form derivatives like 2-alkynyladenosine (Fig. 1) .
-
Ribose modifications : The 5'-OH group participates in phosphorylation reactions using tert-butyl N,N-diisopropylphosphoramidite, yielding analogs such as 1-deoxy-2,3-O-isopropylidene-d-ribofuranose-5-O-di-tert-butylphosphate .
Key reagents :
Reaction Type | Reagents/Conditions | Products |
---|---|---|
C6 substitution | Pd catalysts, alkynes | 2-Alkynyladenosine derivatives |
Ribose phosphorylation | tert-Butyl phosphoramidite, DMF | 5'-Phosphorylated analogs |
Oxidation and Reduction
The ribose hydroxyl groups and purine base are redox-active:
-
Oxidation : Hydroxyl groups at C2' and C3' oxidize to ketones or aldehydes under controlled conditions (e.g., KMnO₄).
-
Reduction : Sodium borohydride reduces oxidized intermediates to stabilize derivatives for NMR studies .
Experimental findings :
-
Oxidation kinetics are pH-dependent, with optimal yields at pH 7–9 .
-
Isotopic labeling at C5' minimally alters redox potentials compared to unlabeled adenosine .
Hydrolysis and Phosphoryl Transfer
This compound participates in enzymatic and nonenzymatic hydrolysis:
-
5'-Nucleotidase-catalyzed hydrolysis : Cleaves the phosphate group to yield adenosine-13C and inorganic phosphate (k = 3.0 × 10⁻¹¹ M⁻¹s⁻¹ at 25°C) .
-
Phosphoryl transfer : ATP analogs undergo Mg²⁺-dependent reactions, with kinetic isotope effects (KIEs) for ¹³C-labeled compounds showing a 1.02–1.05 KIE .
Kinetic parameters :
Substrate | Reaction | Rate Constant (25°C) |
---|---|---|
This compound | Hydrolysis (5'-nucleotidase) | 3.0 × 10⁻¹¹ M⁻¹s⁻¹ |
ATP-¹³C | Phosphoryl transfer (LDH) | KIE = 1.03–1.05 |
Stacking and Self-Association
13C NMR studies reveal concentration-dependent stacking behavior:
-
Self-association : At >50 mM, this compound forms π-stacked dimers, evidenced by upfield ¹³C chemical shifts (Δδ = −1.45 ppm for C8) .
-
Tryptamine complexation : Stacking with tryptamine induces a Δδ of −1.23 ppm at C2, stabilizing charge-transfer complexes .
NMR parameters :
Nucleus | Shift (ppm) | Δδ (Self-association) | Δδ (Tryptamine complex) |
---|---|---|---|
C2 | 153.75 | −1.25 | −1.45 |
C8 | 141.30 | −1.45 | −0.74 |
Enzymatic Modifications
-
Adenosine deaminase : Converts this compound to inosine-13C (kₐₜₖ = 4.5 × 10³ M⁻¹s⁻¹) .
-
Kinase-mediated phosphorylation : ATP synthase incorporates ¹³C into ATP via Mg²⁺-dependent mechanisms, confirmed by ³¹P NMR .
Environmental and Kinetic Influences
-
Temperature : Rotational correlation times (τc) for this compound increase by 30% at 10°C vs. 25°C, slowing reaction rates .
-
Crowding agents : Polyethylene glycol (PEG) reduces diffusion coefficients by 15%, altering reaction equilibria .
Diffusion data :
Condition | Diffusion Coefficient (10⁻¹⁰ m²/s) |
---|---|
10 mM (no PEG) | 4.2 ± 0.1 |
10 mM (20% PEG) | 3.6 ± 0.1 |
Applications De Recherche Scientifique
Chemistry
In chemistry, adenosine-5'-13C is used as a precursor for the synthesis of other nucleoside analogs. It serves as a model compound for studying nucleoside chemistry and reaction mechanisms.
Biology
In biology, this compound is used to study the structure and function of nucleic acids. It helps in understanding the interactions between nucleosides and enzymes involved in DNA and RNA synthesis.
Medicine
In medicine, this compound is investigated for its potential as an antiviral and anticancer agent. Its ability to mimic natural nucleosides allows it to interfere with viral replication and cancer cell proliferation.
Industry
In the pharmaceutical industry, this compound is used in the development of nucleoside-based drugs. It is also employed in the production of diagnostic reagents and molecular probes.
Mécanisme D'action
The mechanism of action of adenosine-5'-13C involves its incorporation into nucleic acids. Once incorporated, it can disrupt normal DNA and RNA synthesis, leading to the inhibition of viral replication or cancer cell growth. The compound targets enzymes like DNA polymerase and reverse transcriptase, which are essential for nucleic acid synthesis.
Comparaison Avec Des Composés Similaires
Comparative Analysis with Structurally Similar Compounds
Adenosine Phosphate Derivatives
Adenosine-5'-triphosphate (ATP), adenosine-5'-diphosphate (ADP), and adenosine-5'-monophosphate (AMP) share the adenosine backbone but differ in phosphate group number. Key comparisons include:
Functional Insights :
- ATP, ADP, and AMP are critical in energy metabolism, whereas this compound serves as a tracer for these pathways .
Sulfate-Reducing Adenosine Derivatives
Adenosine-5'-phosphosulfate (APS) is a key intermediate in sulfate assimilation. Unlike this compound, APS participates in enzymatic reactions catalyzed by APS reductase, with kinetic activity varying across bacterial strains (e.g., 10-fold higher substrate affinity in environmental vs. intestinal sulfate-reducing bacteria) . This functional specialization contrasts with this compound’s role as a passive tracer.
Comparison with Isotopic Variants
Other 13C-labeled adenosine derivatives include:
Key Findings :
- Positional labeling (e.g., 5' vs. 1') affects resolution in NMR studies. For example, 5'-13C labels are optimal for studying phosphorylation events .
- Adenosine-13C5 provides holistic tracing but lacks site-specificity compared to this compound .
Functional Analogues in Drug Discovery
Adenosine-5'-propylphosphate, a synthetic analogue, inhibits acetyl-CoA synthetase (ACSS2) by binding to its nucleotide pocket, as shown in docking studies (PDB: 1PG4) .
Activité Biologique
Adenosine-5'-13C is a carbon-13 isotopically labeled derivative of adenosine, a crucial nucleoside involved in various biological processes. This compound has gained attention in biochemical and pharmacological research due to its potential roles in cellular signaling, metabolism, and therapeutic applications. This article explores the biological activity of this compound, highlighting its mechanisms of action, effects on different biological systems, and relevant case studies.
1. Purinergic Signaling
This compound participates in purinergic signaling pathways, interacting with specific receptors (A1, A2A, A2B, and A3) that mediate various physiological effects. These receptors are involved in processes such as:
- Cardiovascular Regulation : Adenosine acts as a vasodilator and modulates heart rate through A1 receptor activation.
- Neurotransmission : It influences neurotransmitter release and neuronal excitability via A2A receptors.
- Immune Response : Adenosine modulates immune cell function, promoting anti-inflammatory effects through its receptors.
2. Metabolic Effects
This compound is implicated in cellular energy metabolism. It can enhance ATP production through the following mechanisms:
- AMPK Activation : Adenosine activates AMP-activated protein kinase (AMPK), a critical regulator of cellular energy homeostasis.
- Mitochondrial Function : It promotes mitochondrial biogenesis and function, contributing to improved energy metabolism in cells.
Table 1: Biological Activities of this compound
Case Study 1: Cancer Treatment
In a study investigating the effects of adenosine-5'-triphosphate (ATP) infusions in cancer patients, it was found that adenosine derivatives could improve quality of life and functional status by modulating tumor microenvironments and enhancing drug delivery to tumor sites. The study highlighted the potential for adenosine analogs to act as adjunct therapies in cancer treatment by improving the efficacy of chemotherapeutic agents .
Case Study 2: Neurological Disorders
Research has shown that adenosine plays a protective role in neurodegenerative diseases. In animal models of Alzheimer's disease, administration of adenosine derivatives demonstrated neuroprotective effects by reducing neuroinflammation and promoting neuronal survival. This suggests that this compound may have therapeutic potential in treating neurological disorders .
Case Study 3: Cardiovascular Health
A clinical trial assessed the impact of adenosine on patients with cardiovascular diseases. The results indicated that treatment with adenosine derivatives improved endothelial function and reduced ischemic events, underscoring the compound's role in cardiovascular health management .
Q & A
Basic Research Questions
Q. How should adenosine-5'-¹³C be handled and stored to ensure isotopic integrity during metabolic flux studies?
- Methodological Answer : Store adenosine-5'-¹³C at ≤-20°C in airtight, light-protected containers to prevent degradation. Avoid repeated freeze-thaw cycles, as temperature fluctuations can compromise isotopic stability. Use pre-chilled tools during aliquoting to minimize thermal stress. Confirm storage conditions via HPLC or mass spectrometry to verify isotopic purity post-storage .
Q. What experimental controls are critical when using adenosine-5'-¹³C to study nucleotide metabolism in cell cultures?
- Methodological Answer : Include unlabeled adenosine controls to distinguish background metabolic activity from ¹³C incorporation. Use negative controls (e.g., ATP-depleted cell systems) to validate enzyme-specific labeling. Quantify intracellular ATP/ADP ratios via LC-MS to ensure isotopic equilibrium before sampling .
Q. How can researchers confirm the isotopic labeling efficiency of adenosine-5'-¹³C in synthesized samples?
- Methodological Answer : Employ ¹³C-NMR to verify the position-specific enrichment at the 5'-carbon. For high-throughput validation, use LC-HRMS with a mass accuracy threshold of <2 ppm. Compare observed isotopic patterns (e.g., M+1 peaks) against theoretical distributions to calculate labeling efficiency .
Advanced Research Questions
Q. How can adenosine-5'-¹³C be integrated into dynamic ¹³C metabolic flux analysis (MFA) to resolve conflicting data on glycolytic vs. mitochondrial ATP synthesis?
- Methodological Answer : Combine time-resolved isotopic labeling with compartmentalized metabolic modeling. Use parallel labeling experiments with [U-¹³C]-glucose to trace carbon transitions into ATP. Apply statistical flux balance analysis (e.g., Monte Carlo sampling) to reconcile discrepancies between extracellular flux measurements and intracellular ATP labeling kinetics .
Q. What strategies mitigate isotopic dilution effects when studying kinase activity with adenosine-5'-¹³C in heterogeneous cell populations?
- Methodological Answer : Pre-equilibrate cells in isotope-free media to deplete endogenous ATP pools. Use kinetic modeling (e.g., Michaelis-Menten frameworks) adjusted for isotopic dilution. Validate via siRNA knockdown of ATP-consuming pathways (e.g., mTOR signaling) to isolate kinase-specific ¹³C incorporation .
Q. How does adenosine-5'-¹³C enable tracing of microbial methanogenesis pathways in anaerobic sediment environments?
- Methodological Answer : Co-inject adenosine-5'-¹³C with ¹³CH₄ precursors (e.g., acetate-¹³C) into sediment microcosms. Analyze δ¹³C signatures of CH₄ and ATP-derived biomarkers (e.g., branched fatty acids) via GC-IRMS. Correlate isotopic enrichment with ATP cell counts to distinguish acetoclastic vs. hydrogenotrophic methanogenesis .
Q. What analytical approaches resolve contradictions between ¹³C-labeling data and transcriptomic profiles in cancer cell metabolism studies?
- Methodological Answer : Apply multi-omics integration (e.g., isotope-resolved metabolomics coupled with RNA-seq) to identify post-transcriptional regulation. Use ANOVA to test for discrepancies in metabolite vs. gene expression correlations (e.g., p-values <0.05 for TCA cycle enzymes). Normalize ¹³C data to cell-cycle phase using flow cytometry .
Q. How is adenosine-5'-¹³C used to detect AMPylation in post-translational modification studies?
- Methodological Answer : Incubate target proteins with adenosine-5'-¹³C and N6-propargyl-ATP. Enrich AMPylated peptides via click chemistry (CuAAC) and analyze via LC-MS/MS. Identify ¹³C-specific mass shifts (Δm/z = +1.0034) to distinguish endogenous AMPylation from background noise .
Q. What statistical methods are optimal for analyzing dose-response relationships in adenosine-5'-¹³C toxicity assays?
- Methodological Answer : Use two-way ANOVA to assess interactions between isotopic labeling and cytotoxicity (e.g., IC₅₀ values). Apply Tukey’s HSD post hoc test to compare labeled vs. unlabeled adenosine effects. Report effect sizes (η²) to quantify variance explained by isotopic modification .
Q. How can long-term stability studies of adenosine-5'-¹³C be designed to comply with ISO guidelines for isotopic pharmaceuticals?
- Methodological Answer : Conduct accelerated stability testing at 40°C/75% RH for 6 months, with periodic sampling. Analyze degradation products (e.g., deaminated inosine) via UPLC-UV and confirm isotopic integrity via ¹³C-NMR. Validate shelf-life predictions using Arrhenius kinetic modeling .
Q. Data Presentation Guidelines
- Tables : Include isotopic enrichment ratios (e.g., ¹³C/(¹²C+¹³C)) with standard deviations.
- Figures : Use heatmaps for flux distributions or Sankey diagrams for carbon transitions. Avoid overcrowding with chemical structures .
- Statistical Reporting : Provide ANOVA tables with F-statistics, degrees of freedom, and p-values. Use asterisks (*p<0.05, **p<0.01) for significance .
Propriétés
IUPAC Name |
(2R,3R,4S,5R)-2-(6-aminopurin-9-yl)-5-(hydroxy(113C)methyl)oxolane-3,4-diol | |
---|---|---|
Source | PubChem | |
URL | https://pubchem.ncbi.nlm.nih.gov | |
Description | Data deposited in or computed by PubChem | |
InChI |
InChI=1S/C10H13N5O4/c11-8-5-9(13-2-12-8)15(3-14-5)10-7(18)6(17)4(1-16)19-10/h2-4,6-7,10,16-18H,1H2,(H2,11,12,13)/t4-,6-,7-,10-/m1/s1/i1+1 | |
Source | PubChem | |
URL | https://pubchem.ncbi.nlm.nih.gov | |
Description | Data deposited in or computed by PubChem | |
InChI Key |
OIRDTQYFTABQOQ-XUZOCFOZSA-N | |
Source | PubChem | |
URL | https://pubchem.ncbi.nlm.nih.gov | |
Description | Data deposited in or computed by PubChem | |
Canonical SMILES |
C1=NC(=C2C(=N1)N(C=N2)C3C(C(C(O3)CO)O)O)N | |
Source | PubChem | |
URL | https://pubchem.ncbi.nlm.nih.gov | |
Description | Data deposited in or computed by PubChem | |
Isomeric SMILES |
C1=NC(=C2C(=N1)N(C=N2)[C@H]3[C@@H]([C@@H]([C@H](O3)[13CH2]O)O)O)N | |
Source | PubChem | |
URL | https://pubchem.ncbi.nlm.nih.gov | |
Description | Data deposited in or computed by PubChem | |
Molecular Formula |
C10H13N5O4 | |
Source | PubChem | |
URL | https://pubchem.ncbi.nlm.nih.gov | |
Description | Data deposited in or computed by PubChem | |
Molecular Weight |
268.23 g/mol | |
Source | PubChem | |
URL | https://pubchem.ncbi.nlm.nih.gov | |
Description | Data deposited in or computed by PubChem | |
Avertissement et informations sur les produits de recherche in vitro
Veuillez noter que tous les articles et informations sur les produits présentés sur BenchChem sont destinés uniquement à des fins informatives. Les produits disponibles à l'achat sur BenchChem sont spécifiquement conçus pour des études in vitro, qui sont réalisées en dehors des organismes vivants. Les études in vitro, dérivées du terme latin "in verre", impliquent des expériences réalisées dans des environnements de laboratoire contrôlés à l'aide de cellules ou de tissus. Il est important de noter que ces produits ne sont pas classés comme médicaments et n'ont pas reçu l'approbation de la FDA pour la prévention, le traitement ou la guérison de toute condition médicale, affection ou maladie. Nous devons souligner que toute forme d'introduction corporelle de ces produits chez les humains ou les animaux est strictement interdite par la loi. Il est essentiel de respecter ces directives pour assurer la conformité aux normes légales et éthiques en matière de recherche et d'expérimentation.