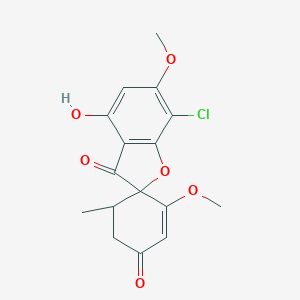
Desmethylgriseofulvin
- Cliquez sur DEMANDE RAPIDE pour recevoir un devis de notre équipe d'experts.
- Avec des produits de qualité à un prix COMPÉTITIF, vous pouvez vous concentrer davantage sur votre recherche.
Vue d'ensemble
Description
Desmethylgriseofulvin is a derivative of griseofulvin, an antifungal polyketide metabolite produced mainly by ascomycetes. Griseofulvin has been used since 1959 to treat dermatophyte infections. This compound, specifically, is a metabolite formed during the biotransformation of griseofulvin in the liver.
Méthodes De Préparation
Synthetic Routes and Reaction Conditions: Desmethylgriseofulvin can be synthesized through the demethylation of griseofulvin. This process typically involves the use of demethylating agents such as boron tribromide (BBr3) or aluminum chloride (AlCl3) under controlled conditions. The reaction is carried out in an inert atmosphere, often using solvents like dichloromethane or chloroform .
Industrial Production Methods: Industrial production of this compound involves the fermentation of specific strains of fungi, such as Penicillium griseofulvum, followed by extraction and purification processes. The fermentation conditions, including temperature, pH, and nutrient supply, are optimized to maximize the yield of this compound .
Analyse Des Réactions Chimiques
Types of Reactions: Desmethylgriseofulvin undergoes various chemical reactions, including:
Oxidation: It can be oxidized to form hydroxylated derivatives.
Reduction: Reduction reactions can convert it into more reduced forms.
Substitution: It can undergo nucleophilic substitution reactions, where functional groups are replaced by nucleophiles.
Common Reagents and Conditions:
Oxidation: Common oxidizing agents include potassium permanganate (KMnO4) and chromium trioxide (CrO3).
Reduction: Reducing agents such as sodium borohydride (NaBH4) or lithium aluminum hydride (LiAlH4) are used.
Substitution: Nucleophiles like sodium methoxide (NaOCH3) or potassium cyanide (KCN) are employed.
Major Products: The major products formed from these reactions include hydroxylated derivatives, reduced forms, and substituted compounds with various functional groups .
Applications De Recherche Scientifique
Chemistry: It is used as a precursor for synthesizing other bioactive compounds.
Biology: Studies have shown its potential in disrupting mitosis and cell division, similar to griseofulvin.
Medicine: It has been investigated for its antifungal properties and potential anticancer activities.
Mécanisme D'action
Desmethylgriseofulvin exerts its effects by binding to microtubules, thereby interfering with microtubule function and inhibiting mitosis. This mechanism is similar to that of griseofulvin. It binds to tubulin, altering the dynamics of microtubules and preventing the proper formation of the mitotic spindle, which is essential for cell division . Additionally, it has been shown to interact with cytokeratin intermediate filament proteins, potentially contributing to its biological effects .
Comparaison Avec Des Composés Similaires
Griseofulvin: The parent compound, known for its antifungal properties.
6-Desmethylgriseofulvin: Another metabolite of griseofulvin with similar biological activities.
Brominated Griseofulvin Derivatives: These compounds have been synthesized to enhance antifungal activity.
Uniqueness: Desmethylgriseofulvin is unique due to its specific demethylation, which alters its biological activity compared to griseofulvin.
Activité Biologique
Desmethylgriseofulvin, a metabolite of the antifungal agent griseofulvin, has garnered attention for its diverse biological activities. This article explores the compound's mechanisms of action, pharmacological effects, and potential therapeutic applications, supported by data from various studies.
Overview of this compound
This compound refers primarily to two metabolites: 6-desmethylgriseofulvin (6-DMG) and 4-desmethylgriseofulvin (4-DMG) . These compounds result from the metabolic breakdown of griseofulvin, which is produced by certain fungi and has been used clinically since the 1950s to treat dermatophyte infections. Recent research has expanded the understanding of its biological activity beyond antifungal properties.
- Antifungal Activity :
- Cancer Cell Inhibition :
- Viral Replication Inhibition :
- Enhancement of ACE2 Function :
Pharmacokinetics
This compound is primarily excreted through urine and has been observed to have varying biological half-lives depending on the individual and dosage. In studies involving mice, significant amounts of 6-DMG and 4-DMG were detected post-administration, indicating active metabolism and excretion pathways .
Case Study: Carcinogenicity Concerns
A cohort study involving over 140,000 individuals indicated a potential link between griseofulvin use and an increased incidence of thyroid cancer; however, no significant associations were found for other cancer types over extended follow-up periods . This raises questions about the long-term safety of this compound metabolites.
Research Findings Table
Binding Affinity Studies
Recent molecular docking analyses revealed that this compound derivatives exhibit strong binding affinities to cytokeratin intermediate filament proteins (K8 and K18), which may play a role in liver injury mechanisms associated with griseofulvin treatment. The binding energies ranged from -3.34 to -5.61 kcal/mol, indicating favorable interactions that could explain observed hepatotoxic effects in animal models .
Propriétés
Numéro CAS |
5128-41-6 |
---|---|
Formule moléculaire |
C16H15ClO6 |
Poids moléculaire |
338.74 g/mol |
Nom IUPAC |
(2S,5'R)-7-chloro-4-hydroxy-3',6-dimethoxy-5'-methylspiro[1-benzofuran-2,4'-cyclohex-2-ene]-1',3-dione |
InChI |
InChI=1S/C16H15ClO6/c1-7-4-8(18)5-11(22-3)16(7)15(20)12-9(19)6-10(21-2)13(17)14(12)23-16/h5-7,19H,4H2,1-3H3/t7-,16+/m1/s1 |
Clé InChI |
KTICLSBYPHKDMM-QZTNRIJFSA-N |
SMILES |
CC1CC(=O)C=C(C12C(=O)C3=C(O2)C(=C(C=C3O)OC)Cl)OC |
SMILES isomérique |
C[C@@H]1CC(=O)C=C([C@]12C(=O)C3=C(O2)C(=C(C=C3O)OC)Cl)OC |
SMILES canonique |
CC1CC(=O)C=C(C12C(=O)C3=C(O2)C(=C(C=C3O)OC)Cl)OC |
Synonymes |
(1’S,6’R)-7-Chloro-4-hydroxy-2’,6-dimethoxy-6’-methylspiro[benzofuran-2(3H),1’-[2]cyclohexene]-3,4’-dione; (1’S-trans)- 7-Chloro-4-hydroxy-2’,6-dimethoxy-6’-methylspiro_x000B_[benzofuran-2(3H),1’-[2]cyclohexene]-3,4’-dione; 4-Desmethylgriseofulvin; |
Origine du produit |
United States |
Retrosynthesis Analysis
AI-Powered Synthesis Planning: Our tool employs the Template_relevance Pistachio, Template_relevance Bkms_metabolic, Template_relevance Pistachio_ringbreaker, Template_relevance Reaxys, Template_relevance Reaxys_biocatalysis model, leveraging a vast database of chemical reactions to predict feasible synthetic routes.
One-Step Synthesis Focus: Specifically designed for one-step synthesis, it provides concise and direct routes for your target compounds, streamlining the synthesis process.
Accurate Predictions: Utilizing the extensive PISTACHIO, BKMS_METABOLIC, PISTACHIO_RINGBREAKER, REAXYS, REAXYS_BIOCATALYSIS database, our tool offers high-accuracy predictions, reflecting the latest in chemical research and data.
Strategy Settings
Precursor scoring | Relevance Heuristic |
---|---|
Min. plausibility | 0.01 |
Model | Template_relevance |
Template Set | Pistachio/Bkms_metabolic/Pistachio_ringbreaker/Reaxys/Reaxys_biocatalysis |
Top-N result to add to graph | 6 |
Feasible Synthetic Routes
Q1: What are the main metabolic pathways of griseofulvin in humans?
A1: In humans, griseofulvin is primarily metabolized in the liver by microsomal enzymes into its main metabolite, 6-desmethylgriseofulvin. [] This metabolite, along with a small amount of griseofulvin itself, 4-desmethylgriseofulvin, and other minor metabolites, are excreted in the urine. [] Interestingly, 6-desmethylgriseofulvin is found both in its free form and as a glucuronide conjugate in the urine. []
Q2: How does the route of administration affect the absorption of griseofulvin?
A2: Research shows that the formulation of griseofulvin significantly impacts its absorption. A corn oil-in-water emulsion containing micronized griseofulvin led to significantly higher absorption rates compared to aqueous suspensions or commercial tablet formulations. [] This enhanced absorption is likely due to the linoleic and oleic acids present in corn oil, which may inhibit gastrointestinal motility and stimulate gallbladder evacuation, thereby improving drug absorption. []
Q3: What is the mechanism of action of griseofulvin and its derivatives against fungi?
A3: Griseofulvin exerts its antifungal activity by binding to tubulin, a protein crucial for the assembly of the mitotic spindle. [] This interaction disrupts the formation of the mitotic spindle, thereby inhibiting mitosis in susceptible fungi, making griseofulvin a fungistatic agent. []
Q4: Besides its antifungal activity, are there other potential therapeutic applications being explored for griseofulvin?
A4: Yes, research suggests that griseofulvin may have other therapeutic applications beyond its antifungal properties. For instance, studies have shown it can disrupt mitosis and cell division in human cancer cells and hinder the replication of the hepatitis C virus. [] Furthermore, there is evidence suggesting that griseofulvin might enhance ACE2 function, contribute to vascular vasodilation, and improve capillary blood flow. []
Q5: Are there any in vitro models used to study the potential cardioprotective effects of griseofulvin?
A5: Yes, research has explored the cardioprotective potential of griseofulvin using an in vitro model of S. aureus-induced infectious myocarditis. [] This model demonstrated that griseofulvin, alongside other compounds like sajaroketide A and altechromone A, exhibited significant cardioprotective effects. []
Q6: How do the metabolic profiles of griseofulvin compare across different model systems?
A6: Studies comparing the metabolism of radiolabeled griseofulvin in rat liver microsomes, isolated perfused rat livers, and rats with bile duct cannulas show consistent results. [] The primary metabolites identified in all three systems were 4-desmethylgriseofulvin and 6-desmethylgriseofulvin. [] Importantly, the ratio of these two metabolites remained relatively consistent across the different models, suggesting a good correlation between in vitro and in vivo metabolism of griseofulvin. []
Avertissement et informations sur les produits de recherche in vitro
Veuillez noter que tous les articles et informations sur les produits présentés sur BenchChem sont destinés uniquement à des fins informatives. Les produits disponibles à l'achat sur BenchChem sont spécifiquement conçus pour des études in vitro, qui sont réalisées en dehors des organismes vivants. Les études in vitro, dérivées du terme latin "in verre", impliquent des expériences réalisées dans des environnements de laboratoire contrôlés à l'aide de cellules ou de tissus. Il est important de noter que ces produits ne sont pas classés comme médicaments et n'ont pas reçu l'approbation de la FDA pour la prévention, le traitement ou la guérison de toute condition médicale, affection ou maladie. Nous devons souligner que toute forme d'introduction corporelle de ces produits chez les humains ou les animaux est strictement interdite par la loi. Il est essentiel de respecter ces directives pour assurer la conformité aux normes légales et éthiques en matière de recherche et d'expérimentation.