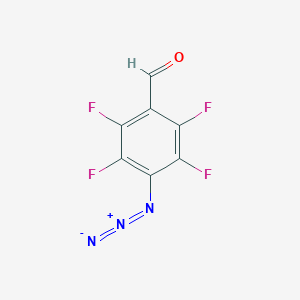
4-Azido-2,3,5,6-tetrafluorobenzaldehyde
Vue d'ensemble
Description
4-Azido-2,3,5,6-tetrafluorobenzaldehyde is a chemical compound that has gained significant attention in scientific research due to its unique chemical structure and potential biological activity. It is a crosslinkable agent with a photoactivable group that can be used in the study of polymers .
Synthesis Analysis
The synthesis of 4-Azido-2,3,5,6-tetrafluorobenzaldehyde can be achieved by nitrosation of 2,3,5,6-tetrafluoro-4-hydrazinopyridine or, preferably, treatment of pentafluoropyridine with sodium azide in acetonitrile .Molecular Structure Analysis
The molecular formula of 4-Azido-2,3,5,6-tetrafluorobenzaldehyde is C7HF4N3O2 . The SMILES string representation is OC(=O)c1c(F)c(F)c(N=[N+]=[N-])c(F)c1F .Chemical Reactions Analysis
4-Azido-2,3,5,6-tetrafluorobenzaldehyde can react with various compounds. For instance, it can undergo the Staudinger reaction with triphenylphosphine at room temperature . It also partakes in 1,3-dipolar cycloaddition reactions with benzyne, diphenylacetylene, phenylacetylene, cyclopentadiene dimer, and norbornene .Applications De Recherche Scientifique
Photolysis to Create Stable Room Temperature Nitrenes
One of the notable applications of 4-Azido compounds is in the creation of stable room temperature nitrenes through photolysis. The photolysis of crystalline 4-Azido-2,3,5,6-tetrafluorobenzoic acid, a closely related compound, has been studied for its ability to form nitrenes that are stable at room temperature . This process has significant implications for materials science and engineering, particularly in the development of new materials with unique properties.
Synthesis of Fluorinated Pyridines
The compound is used in the synthesis of various fluorinated pyridines. These pyridines have diverse applications, including as building blocks for pharmaceuticals and agrochemicals. The azide group in 4-Azido-2,3,5,6-tetrafluorobenzaldehyde can undergo thermal reactions to form different pyridine derivatives, which are valuable in synthetic chemistry .
Quantum Dot Patterning
In the field of display technology, 4-Azido-2,3,5,6-tetrafluorobenzaldehyde derivatives are used for multi-color patterning of colloidal quantum dots. This is crucial for creating high-resolution displays. A light-driven ligand crosslinker derived from this compound has been reported to enable solution-based processing methods for quantum dot patterning .
Bioconjugation in Biomedical Research
The azide functionality of 4-Azido-2,3,5,6-tetrafluorobenzaldehyde makes it an excellent candidate for bioconjugation techniques. It serves as a bifunctional biorthogonal tool, allowing for highly efficient conjugation in biological systems without interfering with natural biochemical processes .
Mécanisme D'action
Target of Action
It is known to be used as a multifunctional photo-affinity label to detect biological receptors .
Mode of Action
4-Azido-2,3,5,6-tetrafluorobenzaldehyde interacts with its targets through a process known as photo-affinity labeling . This involves the use of light to create a covalent bond between the 4-Azido-2,3,5,6-tetrafluorobenzaldehyde and its target, allowing for the detection and study of the target .
Biochemical Pathways
As a photo-affinity label, it is likely involved in pathways related to the function and regulation of the biological receptors it targets .
Result of Action
As a photo-affinity label, it allows for the detection and study of biological receptors, which could lead to a better understanding of these receptors’ functions and roles .
Action Environment
It is known that the compound is light-sensitive and should be stored in a freezer . This suggests that light and temperature are important environmental factors that can influence the compound’s stability and efficacy.
Propriétés
IUPAC Name |
4-azido-2,3,5,6-tetrafluorobenzaldehyde | |
---|---|---|
Source | PubChem | |
URL | https://pubchem.ncbi.nlm.nih.gov | |
Description | Data deposited in or computed by PubChem | |
InChI |
InChI=1S/C7HF4N3O/c8-3-2(1-15)4(9)6(11)7(5(3)10)13-14-12/h1H | |
Source | PubChem | |
URL | https://pubchem.ncbi.nlm.nih.gov | |
Description | Data deposited in or computed by PubChem | |
InChI Key |
FYZZJXVVOINZGG-UHFFFAOYSA-N | |
Source | PubChem | |
URL | https://pubchem.ncbi.nlm.nih.gov | |
Description | Data deposited in or computed by PubChem | |
Canonical SMILES |
C(=O)C1=C(C(=C(C(=C1F)F)N=[N+]=[N-])F)F | |
Source | PubChem | |
URL | https://pubchem.ncbi.nlm.nih.gov | |
Description | Data deposited in or computed by PubChem | |
Molecular Formula |
C7HF4N3O | |
Source | PubChem | |
URL | https://pubchem.ncbi.nlm.nih.gov | |
Description | Data deposited in or computed by PubChem | |
Molecular Weight |
219.10 g/mol | |
Source | PubChem | |
URL | https://pubchem.ncbi.nlm.nih.gov | |
Description | Data deposited in or computed by PubChem | |
Retrosynthesis Analysis
AI-Powered Synthesis Planning: Our tool employs the Template_relevance Pistachio, Template_relevance Bkms_metabolic, Template_relevance Pistachio_ringbreaker, Template_relevance Reaxys, Template_relevance Reaxys_biocatalysis model, leveraging a vast database of chemical reactions to predict feasible synthetic routes.
One-Step Synthesis Focus: Specifically designed for one-step synthesis, it provides concise and direct routes for your target compounds, streamlining the synthesis process.
Accurate Predictions: Utilizing the extensive PISTACHIO, BKMS_METABOLIC, PISTACHIO_RINGBREAKER, REAXYS, REAXYS_BIOCATALYSIS database, our tool offers high-accuracy predictions, reflecting the latest in chemical research and data.
Strategy Settings
Precursor scoring | Relevance Heuristic |
---|---|
Min. plausibility | 0.01 |
Model | Template_relevance |
Template Set | Pistachio/Bkms_metabolic/Pistachio_ringbreaker/Reaxys/Reaxys_biocatalysis |
Top-N result to add to graph | 6 |
Feasible Synthetic Routes
Avertissement et informations sur les produits de recherche in vitro
Veuillez noter que tous les articles et informations sur les produits présentés sur BenchChem sont destinés uniquement à des fins informatives. Les produits disponibles à l'achat sur BenchChem sont spécifiquement conçus pour des études in vitro, qui sont réalisées en dehors des organismes vivants. Les études in vitro, dérivées du terme latin "in verre", impliquent des expériences réalisées dans des environnements de laboratoire contrôlés à l'aide de cellules ou de tissus. Il est important de noter que ces produits ne sont pas classés comme médicaments et n'ont pas reçu l'approbation de la FDA pour la prévention, le traitement ou la guérison de toute condition médicale, affection ou maladie. Nous devons souligner que toute forme d'introduction corporelle de ces produits chez les humains ou les animaux est strictement interdite par la loi. Il est essentiel de respecter ces directives pour assurer la conformité aux normes légales et éthiques en matière de recherche et d'expérimentation.