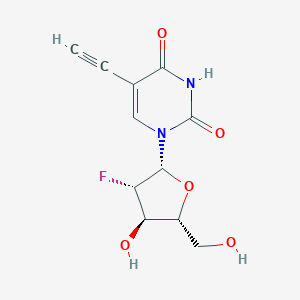
1-(2-Deoxy-2-Fluoro-Beta-D-Arabinofuranosyl)-5-Ethynylpyrimidine-2,4(1h,3h)-Dione
Vue d'ensemble
Description
“1-(2-Deoxy-2-fluoro-beta-D-arabinofuranosyl)uracil” is a compound with the CAS Number: 69123-94-0 . It has been used in trials studying the treatment of Small Intestine Lymphoma, Stage IV Mantle Cell Lymphoma, Waldenström Macroglobulinemia, Splenic Marginal Zone Lymphoma, and Recurrent Mantle Cell Lymphoma, among others .
Molecular Structure Analysis
The molecular formula of this compound is C9H11FN2O5 . The IUPAC name is 1- [ (2 R ,3 S ,4 R ,5 R )-3-fluoro-4-hydroxy-5- (hydroxymethyl)oxolan-2-yl]pyrimidine-2,4-dione . The InChI code is 1S/C9H11FN2O5/c10-6-7 (15)4 (3-13)17-8 (6)12-2-1-5 (14)11-9 (12)16/h1-2,4,6-8,13,15H,3H2, (H,11,14,16)/t4-,6+,7-,8-/m1/s1 .
Physical and Chemical Properties Analysis
The molecular weight of this compound is 246.19 g/mol . The storage temperature is room temperature .
Applications De Recherche Scientifique
Mechanistic Insights and Antiviral Potency
Fluorine substitution in nucleotide analogues, such as the 5-position on the pyrimidine ring, significantly alters kinetic parameters for nucleotide incorporation by HIV-1 reverse transcriptase. This modification enhances the overall efficiency of nucleotide incorporation during DNA and RNA synthesis, potentially explaining the potency of these nucleosides against HIV-1. The steric and electrostatic effects introduced by fluorine substitution are crucial for understanding the drug resistance mechanisms and developing more effective antiviral agents (Ray et al., 2003).
Cancer Treatment and Personalized Medicine
Developments in fluorine chemistry have contributed significantly to the precise use of fluorinated pyrimidines (FPs) in cancer treatment. The review by Gmeiner (2020) emphasizes methods for synthesizing 5-fluorouracil (5-FU) and its impact on nucleic acid structure and dynamics, highlighting the roles of various enzymes in FP cytotoxicity. These insights pave the way for personalized medicine approaches, using polymeric FPs for targeted cancer therapy (Gmeiner, 2020).
Structural and Thermodynamic Basis for Anticancer Activity
The incorporation of antimetabolites, such as fluorinated pyrimidines, into DNA affects its structure and stability. This perturbation has implications for DNA replication and repair, offering a structural and thermodynamic basis for the anticancer activity of antipyrimidines. Understanding these interactions is key for designing effective cancer chemotherapy strategies and novel anticancer drugs (Gmeiner, 2002).
Mécanisme D'action
Target of Action
The primary target of this compound, also known as (2’s)-2’-deoxy-2’-fluoro-5-ethynyluridine, is Thymidylate Synthase . Thymidylate synthase is an enzyme that plays a crucial role in the synthesis of dTMP (deoxythymidine monophosphate), which is essential for DNA replication and repair .
Mode of Action
This compound is a thymidine analog. In several cancer cell lines, it is phosphorylated intracellularly to its monophosphate, 1-(2-deoxy-2-fluoro-beta-D-arabinofuranosyl) uracil monophosphate (FAUMP), by thymidine kinase . It is then methylated in the 5-position by thymidylate synthase to form the product, 1-(2-deoxy-2-fluoro-beta-D-arabinofuranosyl) 5-methyluracil monophosphate (FMAUMP) . This process inhibits the action of thymidylate synthase, thereby disrupting DNA synthesis and leading to cell death .
Biochemical Pathways
The compound affects the DNA synthesis pathway by inhibiting the action of thymidylate synthase . This disruption in the DNA synthesis pathway leads to the inability of the cell to replicate its DNA, which is a critical step in cell division. As a result, the cell cycle is halted, leading to cell death .
Pharmacokinetics
It is known to beCNS penetrant , indicating that it can cross the blood-brain barrier and exert its effects in the central nervous system . More research is needed to fully understand its absorption, distribution, metabolism, and excretion (ADME) properties.
Result of Action
The result of the compound’s action is the inhibition of cell growth and proliferation , particularly in cells with high thymidylate synthase activity . This makes it a potential candidate for the treatment of certain types of cancer .
Safety and Hazards
Orientations Futures
Analyse Biochimique
Biochemical Properties
As a purine nucleoside analogue, 1-(2-Deoxy-2-Fluoro-Beta-D-Arabinofuranosyl)-5-Ethynylpyrimidine-2,4(1h,3h)-Dione can interact with enzymes such as thymidine kinase and thymidylate synthase . These interactions may lead to the inhibition of DNA synthesis and induction of apoptosis , which are key processes in cellular function and survival.
Molecular Mechanism
It is known to interact with enzymes such as thymidine kinase and thymidylate synthase , but the exact nature of these interactions and their consequences at the molecular level are not clear from the search results.
Propriétés
IUPAC Name |
5-ethynyl-1-[(2R,3S,4R,5R)-3-fluoro-4-hydroxy-5-(hydroxymethyl)oxolan-2-yl]pyrimidine-2,4-dione | |
---|---|---|
Source | PubChem | |
URL | https://pubchem.ncbi.nlm.nih.gov | |
Description | Data deposited in or computed by PubChem | |
InChI |
InChI=1S/C11H11FN2O5/c1-2-5-3-14(11(18)13-9(5)17)10-7(12)8(16)6(4-15)19-10/h1,3,6-8,10,15-16H,4H2,(H,13,17,18)/t6-,7+,8-,10-/m1/s1 | |
Source | PubChem | |
URL | https://pubchem.ncbi.nlm.nih.gov | |
Description | Data deposited in or computed by PubChem | |
InChI Key |
YEEGMPUOCRQFRV-IBCQBUCCSA-N | |
Source | PubChem | |
URL | https://pubchem.ncbi.nlm.nih.gov | |
Description | Data deposited in or computed by PubChem | |
Canonical SMILES |
C#CC1=CN(C(=O)NC1=O)C2C(C(C(O2)CO)O)F | |
Source | PubChem | |
URL | https://pubchem.ncbi.nlm.nih.gov | |
Description | Data deposited in or computed by PubChem | |
Isomeric SMILES |
C#CC1=CN(C(=O)NC1=O)[C@H]2[C@H]([C@@H]([C@H](O2)CO)O)F | |
Source | PubChem | |
URL | https://pubchem.ncbi.nlm.nih.gov | |
Description | Data deposited in or computed by PubChem | |
Molecular Formula |
C11H11FN2O5 | |
Source | PubChem | |
URL | https://pubchem.ncbi.nlm.nih.gov | |
Description | Data deposited in or computed by PubChem | |
DSSTOX Substance ID |
DTXSID90914829 | |
Record name | 1-(2-Deoxy-2-fluoropentofuranosyl)-5-ethynyl-4-hydroxypyrimidin-2(1H)-onato | |
Source | EPA DSSTox | |
URL | https://comptox.epa.gov/dashboard/DTXSID90914829 | |
Description | DSSTox provides a high quality public chemistry resource for supporting improved predictive toxicology. | |
Molecular Weight |
270.21 g/mol | |
Source | PubChem | |
URL | https://pubchem.ncbi.nlm.nih.gov | |
Description | Data deposited in or computed by PubChem | |
CAS No. |
95740-26-4 | |
Record name | 5-Ethynyl-1-(3-fluoro-4-hydroxy-5-hydroxymethyl-tetrahydro-furan-2-yl)-1H-pyrimidine-2,4-dione | |
Source | ChemIDplus | |
URL | https://pubchem.ncbi.nlm.nih.gov/substance/?source=chemidplus&sourceid=0095740264 | |
Description | ChemIDplus is a free, web search system that provides access to the structure and nomenclature authority files used for the identification of chemical substances cited in National Library of Medicine (NLM) databases, including the TOXNET system. | |
Record name | 1-(2-Deoxy-2-fluoropentofuranosyl)-5-ethynyl-4-hydroxypyrimidin-2(1H)-onato | |
Source | EPA DSSTox | |
URL | https://comptox.epa.gov/dashboard/DTXSID90914829 | |
Description | DSSTox provides a high quality public chemistry resource for supporting improved predictive toxicology. | |
Q1: How does F-ara-EdU enable DNA labeling, and what are its downstream effects within a cell?
A1: F-ara-EdU is metabolically incorporated into newly synthesized DNA strands during replication. [, ] The ethynyl group in its structure serves as a bioorthogonal handle for copper(I)-catalyzed azide-alkyne cycloaddition ("click" chemistry). This reaction enables the attachment of fluorescent azides to the incorporated F-ara-EdU, thereby allowing visualization and tracking of newly synthesized DNA. [, ] Importantly, unlike other commonly used labels like BrdU or EdU, F-ara-EdU exhibits minimal toxicity and does not significantly perturb cell cycle progression or induce DNA instability. [, ]
Q2: What makes F-ara-EdU advantageous over traditional DNA labeling methods like BrdU for long-term in vivo studies?
A2: F-ara-EdU offers several advantages, particularly in studies requiring prolonged observation of labeled cells. Firstly, it exhibits lower toxicity compared to BrdU and EdU. [, ] This allows researchers to track labeled cells for extended periods without significant cell death or detrimental effects on development, as demonstrated in zebrafish embryo studies. [] Secondly, F-ara-EdU doesn't cause cell cycle arrest, unlike BrdU, making it a more reliable tool for tracking cell proliferation and lineage tracing experiments. []
Q3: Can you elaborate on specific research applications of F-ara-EdU highlighted in the provided scientific papers?
A3: The papers demonstrate F-ara-EdU's utility in several research applications:
- Tracking DNA template strands: Researchers used F-ara-EdU in zebrafish to track the distribution of older DNA strands during cell division. This helped disprove the hypothesis of asymmetric template strand segregation in stem cell niches. []
- Identifying quiescent cells: Using a pulse-chase experiment with F-ara-EdU followed by BrdU labeling, researchers identified populations of slow-cycling or quiescent cells within zebrafish larvae that had incorporated only F-ara-EdU during the initial pulse. []
- Super-resolution imaging: F-ara-EdU, in conjunction with click chemistry and fluorescent probes, is suitable for super-resolution microscopy techniques like structured illumination microscopy (SIM), allowing detailed visualization of chromosome territories and chromatin domains. [, ]
Q4: Are there any limitations to using F-ara-EdU for DNA labeling?
A4: While advantageous, F-ara-EdU might have limitations:
Avertissement et informations sur les produits de recherche in vitro
Veuillez noter que tous les articles et informations sur les produits présentés sur BenchChem sont destinés uniquement à des fins informatives. Les produits disponibles à l'achat sur BenchChem sont spécifiquement conçus pour des études in vitro, qui sont réalisées en dehors des organismes vivants. Les études in vitro, dérivées du terme latin "in verre", impliquent des expériences réalisées dans des environnements de laboratoire contrôlés à l'aide de cellules ou de tissus. Il est important de noter que ces produits ne sont pas classés comme médicaments et n'ont pas reçu l'approbation de la FDA pour la prévention, le traitement ou la guérison de toute condition médicale, affection ou maladie. Nous devons souligner que toute forme d'introduction corporelle de ces produits chez les humains ou les animaux est strictement interdite par la loi. Il est essentiel de respecter ces directives pour assurer la conformité aux normes légales et éthiques en matière de recherche et d'expérimentation.