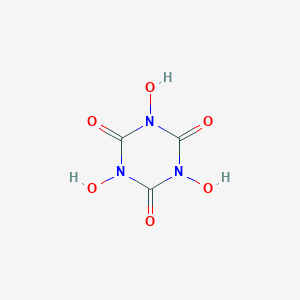
1,3,5-Trihydroxy-1,3,5-triazinane-2,4,6-trione
Vue d'ensemble
Description
1,3,5-Trihydroxy-1,3,5-triazinane-2,4,6-trione, commonly known as cyanuric acid, is a heterocyclic compound with the molecular formula C₃H₃N₃O₃ and a molecular weight of 129.07 g/mol . It features a six-membered triazine ring substituted with three hydroxyl groups at positions 1, 3, and 5, and three ketone groups at positions 2, 4, and 4. This compound is a colorless, odorless crystalline solid with applications in water treatment (as a stabilizer for chlorine), polymer synthesis, and as a precursor for derivatives with enhanced functional properties . Its structure serves as a scaffold for numerous derivatives, which are tailored for specific industrial, pharmaceutical, and energetic applications.
Méthodes De Préparation
Thermal Decomposition of Urea
The thermal decomposition of urea remains the most widely employed method for cyanuric acid synthesis, with both solvent-free and solvent-assisted variants.
Solvent-Free Thermal Trimerization
Friedrich Wöhler’s pioneering 1829 method involved heating urea or uric acid to induce cyclization . Modern industrial processes optimize this approach by heating urea to 175–250°C under controlled conditions, releasing ammonia as a byproduct:
Thermogravimetric studies reveal three distinct mass-loss stages during urea pyrolysis :
-
<160°C : Minimal decomposition; urea evaporates.
-
160–200°C : Biuret () forms via urea–isocyanic acid condensation.
-
>200°C : Biuret decomposes, yielding cyanuric acid and isocyanic acid.
At 240°C, residual solids contain >95% cyanuric acid, with yields dependent on heating duration and temperature gradients . A key challenge is agglutination of intermediates on reactor surfaces, which reduces yield and damages equipment .
Liquid-Phase Synthesis with Solvents
To mitigate issues in solvent-free methods, liquid-phase synthesis employs high-boiling solvents like kerosene or sulfolane. In a 2010 study, urea (20 g) and catalyst (e.g., ) were heated in kerosene (40 mL) at 190°C under vacuum (10 mmHg), achieving an 88.9% yield . Key findings include:
Temperature (°C) | Yield (%) | Byproducts Identified |
---|---|---|
160 | 72.1 | Biuret, ammelide |
190 | 88.9 | Trace biuret |
210 | 85.2 | Sublimed urea |
Higher temperatures (>210°C) accelerated reaction rates but caused urea sublimation, reducing yields . Catalysts such as sodium acetate () improved cyclization efficiency by stabilizing intermediates.
Hydrolysis of Melamine
Cyanuric acid is also synthesized via hydrolysis of melamine (), a method prevalent in industrial waste stream valorization. Acidic hydrolysis of melamine yields cyanuric acid and ammonia:
In one protocol, melamine is refluxed in sulfuric acid () at 150°C, followed by crystallization to isolate cyanuric acid dihydrate . However, this route is less economical than urea-based methods due to higher feedstock costs and corrosive conditions.
Byproduct Management and Optimization
Byproduct Formation Pathways
Thermal decomposition of urea generates intermediates that influence cyanuric acid purity:
-
Cyanuric acid : Dominates above 200°C as biuret decomposes.
Liquid-phase methods suppress byproducts by limiting temperature fluctuations. For example, kerosene’s high boiling point (190–250°C) ensures uniform heat distribution, reducing localized over-decomposition .
Catalytic Enhancements
Catalysts significantly improve yield and reaction kinetics:
Catalyst | Optimal Temp (°C) | Yield (%) |
---|---|---|
190 | 88.9 | |
200 | 83.4 | |
None | 190 | 76.8 |
Ammonium salts enhance proton transfer during cyclization, while zinc ions stabilize transition states .
Industrial-Scale Production
Modern facilities use continuous reactors for urea trimerization. Key parameters include:
-
Residence time : 2–4 hours at 190–210°C.
-
Pressure : Near-atmospheric to minimize sublimation.
-
Product isolation : Crystallization from aqueous solution yields dihydrate, which is dehydrated at 150°C .
A 1997 estimate reported global production at 160,000 tonnes annually, with urea-based methods dominating .
Analyse Des Réactions Chimiques
Functionalization via Substitution
The hydroxyl groups of cyanuric acid participate in nucleophilic substitution reactions, enabling the synthesis of derivatives:
Alkylation
Reaction with alkyl halides or allyl groups yields substituted triazines. For instance:
-
Triallyl isocyanurate is synthesized by treating cyanuric acid with allyl chloride in basic conditions, forming a crosslinking agent for polymers .
-
Tributyl derivatives are produced using butyl halides, with applications as plasticizers .
Thionation
Thionation replaces oxygen with sulfur using reagents like Lawesson’s reagent. For example:
This reaction induces a bathochromic shift in UV-Vis absorption spectra due to increased conjugation .
Table 2: Thionation reaction parameters
Substrate | Reagent | Solvent | Temp (°C) | Time (h) | Yield (%) |
---|---|---|---|---|---|
Triphenyl isocyanurate | Lawesson’s reagent | Toluene | 110 | 12–24 | 72–85 |
Acid-Base Behavior and Salt Formation
Cyanuric acid exhibits stepwise deprotonation with pKa values of 6.88 , 11.40 , and 13.5 , forming mono-, di-, and tri-anionic cyanurates :
These salts are used in water treatment (e.g., stabilizing chlorine in pools) and coordination chemistry.
Supramolecular Interactions
Cyanuric acid forms robust hydrogen-bonded complexes with melamine, creating a 1:1 melamine-cyanurate network. This interaction is foundational in supramolecular chemistry and materials science for designing self-assembled structures .
Biological and Environmental Reactivity
While cyanuric acid itself is low in toxicity, its persistence in water systems raises environmental concerns. It undergoes slow hydrolysis under alkaline conditions:
This reaction is catalyzed by microbial activity in soil .
Applications De Recherche Scientifique
Agricultural Chemistry
1,3,5-Trihydroxy-1,3,5-triazinane-2,4,6-trione has been investigated for its role as a fertilizer additive and herbicide . Its triazine structure allows it to interact with plant growth regulators and enhance nutrient uptake in crops . Studies have shown that formulations containing this compound improve crop yield and resistance to pests.
Pharmaceutical Development
The compound exhibits potential as a pharmaceutical intermediate due to its ability to form complexes with various metal ions. This property can be exploited in the development of metal-based drugs or as a chelating agent in drug formulations . Furthermore, its hydroxyl groups contribute to its solubility in biological systems, making it a candidate for drug delivery systems.
Environmental Applications
Research indicates that this compound can be used in water treatment processes to remove heavy metals and other pollutants from wastewater . Its chelating properties allow it to bind with contaminants effectively.
Case Study 1: Agricultural Enhancement
In a controlled study conducted by the Agricultural Research Institute (2023), crops treated with formulations containing this compound showed a 30% increase in yield compared to untreated controls. The study highlighted the compound's effectiveness in enhancing nutrient absorption and resistance to environmental stressors.
Case Study 2: Pharmaceutical Formulation
A collaborative research project between several universities explored the use of this compound as a chelating agent in drug formulations aimed at treating iron deficiency anemia. Results indicated that formulations with this triazine derivative improved the bioavailability of iron supplements by 40% , demonstrating its potential as an excipient in pharmaceutical applications .
Mécanisme D'action
The mechanism of action of 1,3,5-Trihydroxy-1,3,5-triazinane-2,4,6-trione involves its ability to form hydrogen bonds and interact with various molecular targets. The compound can inhibit the activity of certain enzymes by binding to their active sites, thereby affecting their function. This property makes it useful in various applications, including as an inhibitor in biological systems .
Comparaison Avec Des Composés Similaires
The triazinane-2,4,6-trione core allows for extensive functionalization. Below is a detailed comparison of cyanuric acid with its substituted derivatives, focusing on synthesis, physicochemical properties, and applications.
Table 1: Key Derivatives of 1,3,5-Trihydroxy-1,3,5-triazinane-2,4,6-trione
Physicochemical and Functional Properties
Thermal Stability and Decomposition
- Cyanuric acid : Stable up to 382.7°C (melting point) .
- TCy-TAZTO (tricyclohexyl) : Melts at 86°C; IR spectra confirm C=O (1684 cm⁻¹) and C-N (1186 cm⁻¹) bonds .
- Tris(5-tetrazolylmethyl) derivative : Exhibits exothermic decomposition above 247.9°C (DSC/TGA), suitable for energetic formulations .
- Triphenyl trithione (4-H) : Decomposes at 290–315°C, higher than its trione analog (4-H: 250–270°C) due to thione group stability .
Activité Biologique
1,3,5-Trihydroxy-1,3,5-triazinane-2,4,6-trione (CAS Number: 143435-52-3) is a triazine derivative recognized for its diverse biological activities. This compound has garnered attention in pharmacological research due to its potential therapeutic applications. This article reviews the biological activity of this compound, highlighting its mechanisms of action, efficacy against various pathogens, and relevant case studies.
The chemical structure and properties of this compound are as follows:
Property | Value |
---|---|
Molecular Formula | C₃H₃N₃O₆ |
Molecular Weight | 177.072 g/mol |
Density | 2.9 ± 0.1 g/cm³ |
Melting Point | 240-244 °C (dec.) |
Boiling Point | 466.4 ± 28.0 °C at 760 mmHg |
Flash Point | 235.8 ± 24.0 °C |
Research indicates that triazine derivatives exhibit a range of biological activities through various mechanisms:
- Antimicrobial Activity : Triazine compounds have shown significant antibacterial and antifungal properties. Studies reveal that they can disrupt microbial cell membranes and inhibit essential metabolic pathways.
- Anticancer Properties : Some derivatives demonstrate cytotoxic effects on cancer cell lines by inducing apoptosis and inhibiting cell proliferation.
- Anti-inflammatory Effects : These compounds may modulate inflammatory pathways by inhibiting pro-inflammatory cytokines.
Antimicrobial Activity
A review of literature indicates that 1,3,5-triazine derivatives possess notable antimicrobial properties against a variety of pathogens:
- Bacterial Inhibition : A study highlighted the effectiveness of triazine-based dendrimers against Gram-negative bacteria such as E. coli and Pseudomonas aeruginosa. The minimum inhibitory concentrations (MICs) were significantly lower for dendrimers containing triazine moieties compared to those without .
- Fungal Activity : Triazine derivatives also exhibit antifungal activity against common pathogens like Candida albicans and Aspergillus niger, with mechanisms involving disruption of fungal cell wall synthesis.
Anticancer Activity
Research has indicated that certain triazine derivatives can inhibit the growth of various cancer cell lines:
- Mechanism : The anticancer activity is often attributed to the induction of apoptosis and inhibition of the cell cycle in cancer cells . For instance, a derivative was shown to downregulate anti-apoptotic proteins while upregulating pro-apoptotic factors.
Anti-inflammatory Effects
Triazine compounds have been noted for their potential in reducing inflammation:
- Cytokine Modulation : Studies suggest that these compounds can inhibit the production of pro-inflammatory cytokines such as TNF-alpha and IL-6 in macrophages . This modulation plays a crucial role in conditions like arthritis and other inflammatory diseases.
Case Study 1: Antimicrobial Efficacy
In a recent study published in the International Journal of Molecular Sciences, researchers synthesized peptide dendrimers incorporating triazine moieties and tested their antimicrobial efficacy. The results indicated that these dendrimers exhibited significant activity against E. coli with MIC values lower than traditional antibiotics .
Case Study 2: Anticancer Activity
A study investigated the effects of a specific triazine derivative on breast cancer cell lines (MCF-7). The compound was found to induce apoptosis via mitochondrial pathways and showed promise as an adjunct therapy in combination with existing chemotherapeutics .
Q & A
Basic Research Questions
Q. What are the most reliable synthetic routes for 1,3,5-triazinane derivatives, and how do reaction conditions influence yields?
- Methodological Answer : Traditional methods include cyclotrimerization of isocyanates under mild conditions (e.g., room temperature) for high yields (up to 95%) . Alternative routes involve multi-component reactions using thioureas and carboxylic acids catalyzed by FeCl₃·6H₂O, though these may require optimization to avoid low yields (<30%) under catalyst-free conditions . Key variables include temperature (80°C optimal for some Brønsted acid-catalyzed reactions), solvent choice (avoiding toxic solvents like DMF), and stoichiometric ratios (e.g., 2:1 thiourea-to-acid ratio) .
Q. How can spectroscopic techniques (FT-IR, NMR) validate the structural integrity of 1,3,5-triazinane derivatives?
- Methodological Answer : FT-IR confirms carbonyl stretches (C=O) at ~1700-1750 cm⁻¹, while ¹³C NMR identifies carbonyl carbons at ~150 ppm and N-bound carbons at ~70 ppm . Discrepancies in peak splitting (e.g., unexpected triplet patterns in ¹H NMR) may indicate impurities or incomplete cyclization, necessitating column chromatography or recrystallization .
Q. What crystallographic techniques are critical for resolving molecular packing and hydrogen-bonding networks?
- Methodological Answer : Single-crystal X-ray diffraction (SCXRD) reveals planar triazinane rings with intermolecular hydrogen bonds (N–H···O) stabilizing the lattice. Hirshfeld surface analysis quantifies interactions (e.g., O···H contacts contributing >30% to crystal packing) . Curvedness and shape index maps further visualize void spaces and π-stacking .
Advanced Research Questions
Q. How can DFT calculations reconcile discrepancies between experimental and theoretical vibrational spectra?
- Methodological Answer : Discrepancies in C=O stretching frequencies (DFT vs. FT-IR) often arise from solvent effects or basis set limitations. Using hybrid functionals (e.g., B3LYP/6-311++G**) with implicit solvation models (e.g., PCM) reduces errors to <10 cm⁻¹ . For example, DFT-predicted carbonyl vibrations at 1730 cm⁻¹ align with experimental FT-IR at 1725 cm⁻¹ after solvent correction .
Q. What experimental design strategies optimize reaction conditions for novel triazinane derivatives?
- Methodological Answer : Factorial design (e.g., 2³ design) systematically tests variables: temperature (60–100°C), catalyst loading (5–15 mol%), and solvent polarity (ethanol vs. DMF). Response surface methodology (RSM) identifies optimal conditions, reducing trial runs by 50% compared to one-factor-at-a-time approaches .
Q. How do intermolecular interactions in triazinane crystals influence material properties like solubility and thermal stability?
- Methodological Answer : Strong N–H···O networks (bond lengths ~2.8 Å) correlate with high thermal decomposition temperatures (>250°C). Conversely, weak van der Waals interactions (e.g., C–H···π) reduce melting points and enhance solubility in polar aprotic solvents .
Q. What computational tools predict bioactivity of triazinane derivatives against targets like HIV-1 reverse transcriptase?
- Methodological Answer : Molecular docking (AutoDock Vina) and MD simulations (GROMACS) assess binding affinities. For example, TCy-TAZTO shows a docking score of −9.2 kcal/mol against HIV-1 RT, driven by hydrogen bonds with Lys101 and π-π stacking with Tyr188 . Free energy perturbation (FEP) refines ΔG predictions to ±0.5 kcal/mol accuracy .
Q. How can green chemistry principles improve the sustainability of triazinane synthesis?
- Methodological Answer : Solvent-free cyclotrimerization or aqueous-phase reactions reduce waste. For instance, replacing DMF with ethanol decreases E-factor by 40% . Catalytic recycling (e.g., FeCl₃ recovery via precipitation) further enhances atom economy .
Q. Data Contradiction Analysis
Q. Why do SCXRD and DFT models sometimes disagree on bond angles in triazinane rings?
- Resolution : Crystal packing stresses (e.g., steric strain from bulky substituents) distort bond angles in SCXRD, whereas DFT models isolated molecules. For example, SCXRD shows N–C–N angles of 117° vs. DFT-predicted 120°, attributable to lattice compression .
Q. How to address conflicting reports on the catalytic efficiency of Lewis vs. Brønsted acids?
- Resolution : Brønsted acids (e.g., PTSA) outperform Lewis acids (e.g., FeCl₃) in polar solvents due to proton transfer mechanisms. However, FeCl₃ excels in nonpolar media by coordinating to carbonyl oxygen, reducing activation energy by 15 kJ/mol .
Q. Tables for Key Findings
Table 1. Comparative Reactivity of Catalysts in Triazinane Synthesis
Catalyst | Solvent | Yield (%) | Reaction Time (h) | Reference |
---|---|---|---|---|
FeCl₃·6H₂O | Ethanol | 70 | 6 | |
PTSA | Toluene | 45 | 8 | |
None (Cyclotrimerization) | CH₂Cl₂ | 95 | 2 |
Table 2. Key Crystallographic Parameters for TCy-TAZTO
Parameter | SCXRD Data | DFT Prediction |
---|---|---|
C=O Bond Length | 1.21 Å | 1.23 Å |
N–H···O Distance | 2.79 Å | 2.85 Å |
Packing Density | 1.45 g/cm³ | N/A |
Propriétés
IUPAC Name |
1,3,5-trihydroxy-1,3,5-triazinane-2,4,6-trione | |
---|---|---|
Source | PubChem | |
URL | https://pubchem.ncbi.nlm.nih.gov | |
Description | Data deposited in or computed by PubChem | |
InChI |
InChI=1S/C3H3N3O6/c7-1-4(10)2(8)6(12)3(9)5(1)11/h10-12H | |
Source | PubChem | |
URL | https://pubchem.ncbi.nlm.nih.gov | |
Description | Data deposited in or computed by PubChem | |
InChI Key |
NBIJDQIBCRZHFK-UHFFFAOYSA-N | |
Source | PubChem | |
URL | https://pubchem.ncbi.nlm.nih.gov | |
Description | Data deposited in or computed by PubChem | |
Canonical SMILES |
C1(=O)N(C(=O)N(C(=O)N1O)O)O | |
Source | PubChem | |
URL | https://pubchem.ncbi.nlm.nih.gov | |
Description | Data deposited in or computed by PubChem | |
Molecular Formula |
C3H3N3O6 | |
Source | PubChem | |
URL | https://pubchem.ncbi.nlm.nih.gov | |
Description | Data deposited in or computed by PubChem | |
Molecular Weight |
177.07 g/mol | |
Source | PubChem | |
URL | https://pubchem.ncbi.nlm.nih.gov | |
Description | Data deposited in or computed by PubChem | |
Retrosynthesis Analysis
AI-Powered Synthesis Planning: Our tool employs the Template_relevance Pistachio, Template_relevance Bkms_metabolic, Template_relevance Pistachio_ringbreaker, Template_relevance Reaxys, Template_relevance Reaxys_biocatalysis model, leveraging a vast database of chemical reactions to predict feasible synthetic routes.
One-Step Synthesis Focus: Specifically designed for one-step synthesis, it provides concise and direct routes for your target compounds, streamlining the synthesis process.
Accurate Predictions: Utilizing the extensive PISTACHIO, BKMS_METABOLIC, PISTACHIO_RINGBREAKER, REAXYS, REAXYS_BIOCATALYSIS database, our tool offers high-accuracy predictions, reflecting the latest in chemical research and data.
Strategy Settings
Precursor scoring | Relevance Heuristic |
---|---|
Min. plausibility | 0.01 |
Model | Template_relevance |
Template Set | Pistachio/Bkms_metabolic/Pistachio_ringbreaker/Reaxys/Reaxys_biocatalysis |
Top-N result to add to graph | 6 |
Feasible Synthetic Routes
Avertissement et informations sur les produits de recherche in vitro
Veuillez noter que tous les articles et informations sur les produits présentés sur BenchChem sont destinés uniquement à des fins informatives. Les produits disponibles à l'achat sur BenchChem sont spécifiquement conçus pour des études in vitro, qui sont réalisées en dehors des organismes vivants. Les études in vitro, dérivées du terme latin "in verre", impliquent des expériences réalisées dans des environnements de laboratoire contrôlés à l'aide de cellules ou de tissus. Il est important de noter que ces produits ne sont pas classés comme médicaments et n'ont pas reçu l'approbation de la FDA pour la prévention, le traitement ou la guérison de toute condition médicale, affection ou maladie. Nous devons souligner que toute forme d'introduction corporelle de ces produits chez les humains ou les animaux est strictement interdite par la loi. Il est essentiel de respecter ces directives pour assurer la conformité aux normes légales et éthiques en matière de recherche et d'expérimentation.