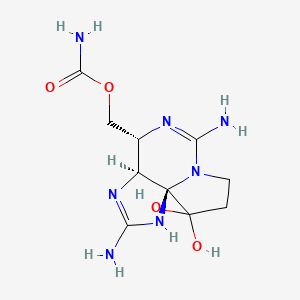
Saxitoxine
Vue d'ensemble
Description
La saxitoxine est une neurotoxine puissante et la toxine paralysante des mollusques la plus connue. Elle est produite par certaines espèces de dinoflagellés marins (tels qu'Alexandrium, Gymnodinium et Pyrodinium) et de cyanobactéries d'eau douce (tels que Dolichospermum, Aphanizomenon, Cylindrospermopsis, Lyngbya et Planktothrix) . La this compound est responsable de la paralysie des mollusques, une maladie grave qui survient lorsque les humains consomment des mollusques contaminés par des proliférations d'algues toxiques .
Applications De Recherche Scientifique
Saxitoxin has numerous scientific research applications due to its potent neurotoxic properties. In chemistry, it is used as a model compound for studying the mechanisms of neurotoxicity and ion channel interactions . In biology, saxitoxin is used to investigate the effects of neurotoxins on cellular processes and to develop assays for detecting toxic algal blooms . In medicine, saxitoxin is being explored for its potential use as a painkiller due to its ability to block sodium channels . Additionally, saxitoxin is used in environmental monitoring to assess the presence of harmful algal blooms in aquatic ecosystems .
Mécanisme D'action
Safety and Hazards
Ingestion of Saxitoxin can cause numbness of the oral mucosa as quickly as 30 minutes after exposure. In severe poisoning, illness typically progresses rapidly and may include gastrointestinal (nausea, vomiting) and neurological (cranial nerve dysfunction, a floating sensation, headache, muscle weakness, parasthesias and vertigo) signs and symptoms. Respiratory failure and death can occur from paralysis .
Orientations Futures
Research is ongoing to better understand the biosynthesis and metabolism of saxitoxin-group toxins in PST-producing organisms, which may potentially contribute to a better strategy in combating harmful algal blooms especially dinoflagellate blooms in the future . Another study suggests that Saxitoxin and tetrodotoxin bioavailability increases in future oceans .
Méthodes De Préparation
Voies de synthèse et conditions de réaction : La synthèse de la saxitoxine est complexe en raison de sa structure moléculaire complexe. Des conditions de réaction spécifiques, telles que la température, le pH et l'utilisation de catalyseurs, sont cruciales pour la synthèse réussie de la this compound .
Méthodes de production industrielle : La production industrielle de la this compound n'est pas courante en raison de sa forte toxicité et de la complexité de sa synthèse. Au lieu de cela, la this compound est généralement extraite de sources naturelles, telles que les mollusques contaminés, à l'aide de méthodes telles que l'extraction liquide-liquide et l'extraction en phase solide .
Analyse Des Réactions Chimiques
Types de réactions : La saxitoxine subit diverses réactions chimiques, notamment l'oxydation, la réduction et la substitution. Ces réactions sont essentielles pour comprendre son comportement et ses interactions avec d'autres composés .
Réactifs et conditions courantes : Les réactifs courants utilisés dans les réactions impliquant la this compound comprennent les agents oxydants, les agents réducteurs et les nucléophiles. Les conditions de réaction, telles que la température, le solvant et le pH, jouent un rôle important dans la détermination du résultat de ces réactions .
Principaux produits : Les principaux produits formés à partir des réactions de la this compound dépendent des conditions de réaction et des réactifs spécifiques utilisés. Par exemple, l'oxydation de la this compound peut conduire à la formation de divers dérivés oxydés .
4. Applications de la recherche scientifique
La this compound possède de nombreuses applications de recherche scientifique en raison de ses propriétés neurotoxiques puissantes. En chimie, elle est utilisée comme composé modèle pour étudier les mécanismes de la neurotoxicité et les interactions avec les canaux ioniques . En biologie, la this compound est utilisée pour étudier les effets des neurotoxines sur les processus cellulaires et pour développer des essais de détection des proliférations d'algues toxiques . En médecine, la this compound est explorée pour son utilisation potentielle comme analgésique en raison de sa capacité à bloquer les canaux sodiques . En outre, la this compound est utilisée dans la surveillance environnementale pour évaluer la présence de proliférations d'algues nocives dans les écosystèmes aquatiques .
5. Mécanisme d'action
La this compound exerce ses effets en bloquant les canaux sodiques dépendants du voltage sur les membranes cellulaires, empêchant l'afflux d'ions sodium dans les cellules . Cette inhibition du passage des ions sodium perturbe la génération et la transmission des potentiels d'action dans les neurones et les cellules musculaires, entraînant une paralysie . Les cibles moléculaires de la this compound comprennent les canaux sodiques du système nerveux périphérique et des muscles squelettiques .
Composés similaires :
- Neothis compound
- Gonyautoxines
- Décarylthis compound
- Tétrodotoxine
Comparaison : La this compound est unique parmi ces composés en raison de sa structure moléculaire spécifique et de sa forte puissance en tant que neurotoxine . Bien que la néothis compound et les gonyautoxines partagent des mécanismes d'action similaires, elles diffèrent par leurs structures chimiques et leurs niveaux de toxicité . La tétrodotoxine, bien que chimiquement différente, bloque également les canaux sodiques et produit des effets paralysants similaires .
La particularité de la this compound réside dans sa forte affinité pour les canaux sodiques et sa présence répandue dans les milieux marins et d'eau douce .
Comparaison Avec Des Composés Similaires
- Neosaxitoxin
- Gonyautoxins
- Decarbamoylsaxitoxin
- Tetrodotoxin
Comparison: Saxitoxin is unique among these compounds due to its specific molecular structure and high potency as a neurotoxin . While neosaxitoxin and gonyautoxins share similar mechanisms of action, they differ in their chemical structures and toxicity levels . Tetrodotoxin, although chemically different, also blocks sodium channels and produces similar paralytic effects .
Saxitoxin’s uniqueness lies in its high affinity for sodium channels and its widespread occurrence in marine and freshwater environments .
Propriétés
IUPAC Name |
[(3aS,4R,10aS)-2,6-diamino-10,10-dihydroxy-3a,4,8,9-tetrahydro-1H-pyrrolo[1,2-c]purin-4-yl]methyl carbamate | |
---|---|---|
Source | PubChem | |
URL | https://pubchem.ncbi.nlm.nih.gov | |
Description | Data deposited in or computed by PubChem | |
InChI |
InChI=1S/C10H17N7O4/c11-6-15-5-4(3-21-8(13)18)14-7(12)17-2-1-9(19,20)10(5,17)16-6/h4-5,19-20H,1-3H2,(H2,12,14)(H2,13,18)(H3,11,15,16)/t4-,5-,10-/m0/s1 | |
Source | PubChem | |
URL | https://pubchem.ncbi.nlm.nih.gov | |
Description | Data deposited in or computed by PubChem | |
InChI Key |
RPQXVSUAYFXFJA-HGRQIUPRSA-N | |
Source | PubChem | |
URL | https://pubchem.ncbi.nlm.nih.gov | |
Description | Data deposited in or computed by PubChem | |
Canonical SMILES |
C1CN2C(=NC(C3C2(C1(O)O)NC(=N3)N)COC(=O)N)N | |
Source | PubChem | |
URL | https://pubchem.ncbi.nlm.nih.gov | |
Description | Data deposited in or computed by PubChem | |
Isomeric SMILES |
C1CN2C(=N[C@H]([C@H]3[C@]2(C1(O)O)NC(=N3)N)COC(=O)N)N | |
Source | PubChem | |
URL | https://pubchem.ncbi.nlm.nih.gov | |
Description | Data deposited in or computed by PubChem | |
Molecular Formula |
C10H17N7O4 | |
Source | PubChem | |
URL | https://pubchem.ncbi.nlm.nih.gov | |
Description | Data deposited in or computed by PubChem | |
DSSTOX Substance ID |
DTXSID3074313 | |
Record name | Saxitoxin | |
Source | EPA DSSTox | |
URL | https://comptox.epa.gov/dashboard/DTXSID3074313 | |
Description | DSSTox provides a high quality public chemistry resource for supporting improved predictive toxicology. | |
Molecular Weight |
299.29 g/mol | |
Source | PubChem | |
URL | https://pubchem.ncbi.nlm.nih.gov | |
Description | Data deposited in or computed by PubChem | |
Physical Description |
Solid; [Sigma-Aldrich MSDS] | |
Record name | Saxitoxin | |
Source | Haz-Map, Information on Hazardous Chemicals and Occupational Diseases | |
URL | https://haz-map.com/Agents/21671 | |
Description | Haz-Map® is an occupational health database designed for health and safety professionals and for consumers seeking information about the adverse effects of workplace exposures to chemical and biological agents. | |
Explanation | Copyright (c) 2022 Haz-Map(R). All rights reserved. Unless otherwise indicated, all materials from Haz-Map are copyrighted by Haz-Map(R). No part of these materials, either text or image may be used for any purpose other than for personal use. Therefore, reproduction, modification, storage in a retrieval system or retransmission, in any form or by any means, electronic, mechanical or otherwise, for reasons other than personal use, is strictly prohibited without prior written permission. | |
CAS No. |
35523-89-8, 73603-72-2 | |
Record name | Saxitoxin | |
Source | CAS Common Chemistry | |
URL | https://commonchemistry.cas.org/detail?cas_rn=35523-89-8 | |
Description | CAS Common Chemistry is an open community resource for accessing chemical information. Nearly 500,000 chemical substances from CAS REGISTRY cover areas of community interest, including common and frequently regulated chemicals, and those relevant to high school and undergraduate chemistry classes. This chemical information, curated by our expert scientists, is provided in alignment with our mission as a division of the American Chemical Society. | |
Explanation | The data from CAS Common Chemistry is provided under a CC-BY-NC 4.0 license, unless otherwise stated. | |
Record name | Saxitoxin | |
Source | ChemIDplus | |
URL | https://pubchem.ncbi.nlm.nih.gov/substance/?source=chemidplus&sourceid=0035523898 | |
Description | ChemIDplus is a free, web search system that provides access to the structure and nomenclature authority files used for the identification of chemical substances cited in National Library of Medicine (NLM) databases, including the TOXNET system. | |
Record name | Saxitoxin-t | |
Source | ChemIDplus | |
URL | https://pubchem.ncbi.nlm.nih.gov/substance/?source=chemidplus&sourceid=0073603722 | |
Description | ChemIDplus is a free, web search system that provides access to the structure and nomenclature authority files used for the identification of chemical substances cited in National Library of Medicine (NLM) databases, including the TOXNET system. | |
Record name | Saxitoxin | |
Source | EPA DSSTox | |
URL | https://comptox.epa.gov/dashboard/DTXSID3074313 | |
Description | DSSTox provides a high quality public chemistry resource for supporting improved predictive toxicology. | |
Record name | Saxitoxin | |
Source | European Chemicals Agency (ECHA) | |
URL | https://echa.europa.eu/information-on-chemicals | |
Description | The European Chemicals Agency (ECHA) is an agency of the European Union which is the driving force among regulatory authorities in implementing the EU's groundbreaking chemicals legislation for the benefit of human health and the environment as well as for innovation and competitiveness. | |
Explanation | Use of the information, documents and data from the ECHA website is subject to the terms and conditions of this Legal Notice, and subject to other binding limitations provided for under applicable law, the information, documents and data made available on the ECHA website may be reproduced, distributed and/or used, totally or in part, for non-commercial purposes provided that ECHA is acknowledged as the source: "Source: European Chemicals Agency, http://echa.europa.eu/". Such acknowledgement must be included in each copy of the material. ECHA permits and encourages organisations and individuals to create links to the ECHA website under the following cumulative conditions: Links can only be made to webpages that provide a link to the Legal Notice page. | |
Record name | SAXITOXIN | |
Source | FDA Global Substance Registration System (GSRS) | |
URL | https://gsrs.ncats.nih.gov/ginas/app/beta/substances/Q0638E899B | |
Description | The FDA Global Substance Registration System (GSRS) enables the efficient and accurate exchange of information on what substances are in regulated products. Instead of relying on names, which vary across regulatory domains, countries, and regions, the GSRS knowledge base makes it possible for substances to be defined by standardized, scientific descriptions. | |
Explanation | Unless otherwise noted, the contents of the FDA website (www.fda.gov), both text and graphics, are not copyrighted. They are in the public domain and may be republished, reprinted and otherwise used freely by anyone without the need to obtain permission from FDA. Credit to the U.S. Food and Drug Administration as the source is appreciated but not required. | |
Record name | Saxitoxin | |
Source | Human Metabolome Database (HMDB) | |
URL | http://www.hmdb.ca/metabolites/HMDB0029368 | |
Description | The Human Metabolome Database (HMDB) is a freely available electronic database containing detailed information about small molecule metabolites found in the human body. | |
Explanation | HMDB is offered to the public as a freely available resource. Use and re-distribution of the data, in whole or in part, for commercial purposes requires explicit permission of the authors and explicit acknowledgment of the source material (HMDB) and the original publication (see the HMDB citing page). We ask that users who download significant portions of the database cite the HMDB paper in any resulting publications. | |
Avertissement et informations sur les produits de recherche in vitro
Veuillez noter que tous les articles et informations sur les produits présentés sur BenchChem sont destinés uniquement à des fins informatives. Les produits disponibles à l'achat sur BenchChem sont spécifiquement conçus pour des études in vitro, qui sont réalisées en dehors des organismes vivants. Les études in vitro, dérivées du terme latin "in verre", impliquent des expériences réalisées dans des environnements de laboratoire contrôlés à l'aide de cellules ou de tissus. Il est important de noter que ces produits ne sont pas classés comme médicaments et n'ont pas reçu l'approbation de la FDA pour la prévention, le traitement ou la guérison de toute condition médicale, affection ou maladie. Nous devons souligner que toute forme d'introduction corporelle de ces produits chez les humains ou les animaux est strictement interdite par la loi. Il est essentiel de respecter ces directives pour assurer la conformité aux normes légales et éthiques en matière de recherche et d'expérimentation.