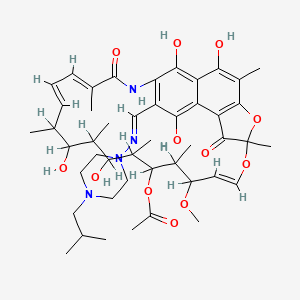
Rifamdin
Vue d'ensemble
Description
Rifampicin belongs to the class of medicines called antibiotics and works to kill or prevent the growth of bacteria . It is used to treat or prevent tuberculosis (TB) and may also be used to reduce certain bacteria in your nose and throat that could cause meningitis or other infections .
Synthesis Analysis
Rifampicin is a first-line hydrophobic anti-tuberculosis drug. An injectable Rifampicin-loaded cement was prepared as a bone scaffold and drug delivery vehicle . The samples were characterized by scanning electron microscope (SEM), Fourier transform infrared (FTIR) spectroscopy, and X-ray diffraction (XRD) .
Molecular Structure Analysis
Rifampicin has a molecular formula of C43H58N4O12 . Its average mass is 822.940 Da and its monoisotopic mass is 822.405151 Da . The molecular structures of the various rifamycins differ by the type and the position of the substituents on the aromatic ring .
Chemical Reactions Analysis
Rifampicin’s solubility and stability were improved as an inclusion complex of acyclic CB[n]. The inclusion complex was prepared by the kneading method, and the physicochemical properties were characterized by spectroscopic techniques .
Physical And Chemical Properties Analysis
Rifampicin has a molecular weight of 822.94 and is soluble in DMSO at 100 mg/mL . It is stored at -20°C for 3 years in powder form and for 6 months at -80°C in solvent .
Applications De Recherche Scientifique
Treatment of Tuberculosis
Rifamdin is one of the first-line drugs against tuberculosis . It has been used for decades in the clinic for the treatment of this disease .
Treatment of Leprosy
Rifamdin is also used in the treatment of leprosy . Its effectiveness against this disease has made it a valuable tool in the fight against this debilitating condition .
Treatment of AIDS-related Mycobacterial Infections
Rifamdin has been used for the treatment of AIDS-related mycobacterial infections . This highlights its broad-spectrum antimicrobial activities .
Treatment of Staphylococcal Implant-Associated Infections
Rifamdin is a potent antibiotic against staphylococcal implant-associated infections . In the absence of implants, current data suggest against the use of rifampin combinations .
Role in Biofilm-Related Infections
Rifamdin is one of the most important biofilm-active antibiotics in the treatment of periprosthetic joint infection (PJI) . Antibiotic regimens not involving rifampin were shown to have higher failure rates .
Study of Antibiotic Resistance
The emergence of rifampin resistance can have a devastating effect on the outcome of PJI . Therefore, Rifamdin is often used in scientific research to study antibiotic resistance .
Mécanisme D'action
Target of Action
Rifamdin, also known as Rifampin, primarily targets the bacterial DNA-dependent RNA polymerase (RNAP) . This enzyme is responsible for DNA transcription in bacteria .
Mode of Action
Rifamdin inhibits the bacterial RNAP by forming a stable drug-enzyme complex . It binds to the beta-subunit of the RNAP , deep within the DNA/RNA channel, thereby blocking the elongation of RNA . This inhibition impedes RNA synthesis and reduces the affinity of RNAP for short RNA transcripts .
Biochemical Pathways
Rifamdin affects several biochemical pathways. It can stimulate the expression of cytochrome P450 (CYP) enzymes by activating the pregnane X receptor (PXR), leading to endoplasmic reticulum (ER) stress and cell apoptosis . Additionally, Rifamdin reduces the expression of the bile salt export pump (BSEP), possibly by suppressing the activity of NRF2, leading to the accumulation of bile acids .
Pharmacokinetics
Rifamdin undergoes rapid and complete absorption after oral administration . Its exposure has considerable inter- and intra-individual variability that could be reduced by administration during fasting . Several factors, including malnutrition, HIV infection, diabetes mellitus, dose size, pharmacogenetic polymorphisms, hepatic cirrhosis, and substandard medicinal products, can alter Rifamdin’s exposure and/or efficacy . Renal impairment has no influence on Rifamdin’s pharmacokinetics when dosed at 600 mg . The maximum concentration (Cmax) of Rifamdin > 8.2 μg/mL is an independent predictor of sterilizing activity .
Result of Action
The primary result of Rifamdin’s action is the inhibition of bacterial RNA synthesis, which effectively halts the growth and proliferation of the bacteria . This makes Rifamdin a potent antibiotic, used alone or in combination with other antimicrobial drugs, to treat tuberculosis and asymptomatic carriers of Neisseria meningitidis .
Action Environment
The action, efficacy, and stability of Rifamdin can be influenced by various environmental factors. For instance, Rifamdin’s absorption is very sensitive to changes in product formulation and is improved when the oral dose is taken on an empty stomach . Furthermore, Rifamdin is a potent enzyme inducer of the cytochrome P450 oxidase system, notably CYP3A4, which can lead to reduced bioavailability and enhanced clearance of some coadministered medications . Therefore, patients receiving any rifamycin should have their medication regimen analyzed carefully for drug interactions .
Safety and Hazards
Rifampicin is considered hazardous by the 2012 OSHA Hazard Communication Standard (29 CFR 1910.1200). It is harmful if swallowed, causes skin irritation, causes serious eye irritation, and may cause respiratory irritation .
Orientations Futures
Propriétés
IUPAC Name |
[(9E,19E,21E)-2,15,17,27,29-pentahydroxy-11-methoxy-3,7,12,14,16,18,22-heptamethyl-26-[(E)-[4-(2-methylpropyl)piperazin-1-yl]iminomethyl]-6,23-dioxo-8,30-dioxa-24-azatetracyclo[23.3.1.14,7.05,28]triaconta-1(29),2,4,9,19,21,25,27-octaen-13-yl] acetate | |
---|---|---|
Source | PubChem | |
URL | https://pubchem.ncbi.nlm.nih.gov | |
Description | Data deposited in or computed by PubChem | |
InChI |
InChI=1S/C46H64N4O12/c1-23(2)22-49-16-18-50(19-17-49)47-21-31-36-41(56)34-33(40(31)55)35-43(29(8)39(34)54)62-46(10,44(35)57)60-20-15-32(59-11)26(5)42(61-30(9)51)28(7)38(53)27(6)37(52)24(3)13-12-14-25(4)45(58)48-36/h12-15,20-21,23-24,26-28,32,37-38,42,52-56H,16-19,22H2,1-11H3,(H,48,58)/b13-12+,20-15+,25-14+,47-21+ | |
Source | PubChem | |
URL | https://pubchem.ncbi.nlm.nih.gov | |
Description | Data deposited in or computed by PubChem | |
InChI Key |
LUSSUGVLLJCTQA-PGNJPLJPSA-N | |
Source | PubChem | |
URL | https://pubchem.ncbi.nlm.nih.gov | |
Description | Data deposited in or computed by PubChem | |
Canonical SMILES |
CC1C=CC=C(C(=O)NC2=C(C(=C3C(=C2O)C(=C(C4=C3C(=O)C(O4)(OC=CC(C(C(C(C(C(C1O)C)O)C)OC(=O)C)C)OC)C)C)O)O)C=NN5CCN(CC5)CC(C)C)C | |
Source | PubChem | |
URL | https://pubchem.ncbi.nlm.nih.gov | |
Description | Data deposited in or computed by PubChem | |
Isomeric SMILES |
CC1/C=C/C=C(/C(=O)NC2=C(C(=C3C(=C2O)C(=C(C4=C3C(=O)C(O4)(O/C=C/C(C(C(C(C(C(C1O)C)O)C)OC(=O)C)C)OC)C)C)O)O)/C=N/N5CCN(CC5)CC(C)C)\C | |
Source | PubChem | |
URL | https://pubchem.ncbi.nlm.nih.gov | |
Description | Data deposited in or computed by PubChem | |
Molecular Formula |
C46H64N4O12 | |
Source | PubChem | |
URL | https://pubchem.ncbi.nlm.nih.gov | |
Description | Data deposited in or computed by PubChem | |
Molecular Weight |
865.0 g/mol | |
Source | PubChem | |
URL | https://pubchem.ncbi.nlm.nih.gov | |
Description | Data deposited in or computed by PubChem | |
Product Name |
Rifamdin | |
CAS RN |
89499-17-2 | |
Record name | Rifamdin | |
Source | ChemIDplus | |
URL | https://pubchem.ncbi.nlm.nih.gov/substance/?source=chemidplus&sourceid=0089499172 | |
Description | ChemIDplus is a free, web search system that provides access to the structure and nomenclature authority files used for the identification of chemical substances cited in National Library of Medicine (NLM) databases, including the TOXNET system. | |
Q & A
Q1: What is the primary mechanism of action of Rifamdin?
A1: Rifamdin, similar to Rifampicin (RFP), exerts its antibacterial effect by inhibiting bacterial DNA-dependent RNA polymerase. [] This inhibition disrupts the crucial process of transcription, effectively preventing bacteria from synthesizing RNA and subsequently, proteins, leading to bacterial death.
Q2: How does Rifamdin resistance develop in bacteria?
A2: Research indicates that bacterial resistance to Rifamdin can arise through two primary mechanisms. The first involves a decreased susceptibility of the bacterial RNA polymerase to Rifamdin. The second mechanism involves alterations in the permeability of the bacterial envelope, hindering the drug's ability to reach its target. [, ]
Q3: Are there specific bacterial species where Rifamdin resistance mechanisms have been studied?
A3: Yes, studies have investigated Rifamdin resistance in Staphylococcus aureus, Escherichia coli, and Proteus vulgaris. Interestingly, while Rifamdin effectively inhibited the RNA polymerase activity in sensitive strains of these bacteria, it did not affect the resistant strains, highlighting the role of altered RNA polymerase sensitivity in resistance. []
Q4: Has Rifamdin's impact on the pharmacokinetics of other drugs been investigated?
A4: Yes, studies in rabbits have explored the influence of Rifamdin on the pharmacokinetics of Dexamethasone. Results showed that pre-treatment with Rifamdin significantly increased the metabolic clearance of Dexamethasone from the plasma. [, ] This suggests a potential drug-drug interaction that might necessitate dosage adjustments when these drugs are co-administered.
Q5: What analytical techniques have been employed to study Rifamdin concentrations?
A5: Research has utilized reversed-phase High-Performance Liquid Chromatography with Ultraviolet detection (HPLC-UV) to analyze Rifamdin concentrations in biological samples. [, ] This technique enables the accurate quantification of the drug, facilitating pharmacokinetic studies and investigations into drug-drug interactions.
Q6: Are there any alternative formulations for Rifamdin?
A6: While traditional formulations exist, research has explored alternative injectable formulations for Rifamdin. These formulations incorporate organic cosolvents, alkaline antioxidants, injection water surfactants, and sodium hydroxide, aiming to improve solubility and potentially enhance its pharmacokinetic profile. []
Avertissement et informations sur les produits de recherche in vitro
Veuillez noter que tous les articles et informations sur les produits présentés sur BenchChem sont destinés uniquement à des fins informatives. Les produits disponibles à l'achat sur BenchChem sont spécifiquement conçus pour des études in vitro, qui sont réalisées en dehors des organismes vivants. Les études in vitro, dérivées du terme latin "in verre", impliquent des expériences réalisées dans des environnements de laboratoire contrôlés à l'aide de cellules ou de tissus. Il est important de noter que ces produits ne sont pas classés comme médicaments et n'ont pas reçu l'approbation de la FDA pour la prévention, le traitement ou la guérison de toute condition médicale, affection ou maladie. Nous devons souligner que toute forme d'introduction corporelle de ces produits chez les humains ou les animaux est strictement interdite par la loi. Il est essentiel de respecter ces directives pour assurer la conformité aux normes légales et éthiques en matière de recherche et d'expérimentation.