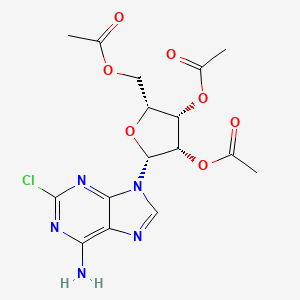
2-Chloro-6-amino-9-(2',3',5'-tri-O-acetyl-beta-D-ribofuranosyl)purine
Vue d'ensemble
Description
2-Chloro-6-amino-9-(2’,3’,5’-tri-O-acetyl-beta-D-ribofuranosyl)purine is a synthetic nucleoside analog. This compound is notable for its structural similarity to naturally occurring nucleosides, making it a valuable tool in biochemical and pharmaceutical research. Its unique structure allows it to interact with biological systems in ways that can be harnessed for therapeutic purposes, particularly in antiviral and anticancer applications.
Méthodes De Préparation
Synthetic Routes and Reaction Conditions
The synthesis of 2-Chloro-6-amino-9-(2’,3’,5’-tri-O-acetyl-beta-D-ribofuranosyl)purine typically involves the following steps:
Starting Materials: The synthesis begins with the preparation of the ribofuranosyl moiety, which is then acetylated to protect the hydroxyl groups.
Nucleoside Formation: The acetylated ribofuranosyl derivative is then coupled with 2-chloro-6-aminopurine under acidic conditions to form the desired nucleoside analog.
Purification: The final product is purified using chromatographic techniques to ensure high purity and yield.
Industrial Production Methods
In an industrial setting, the production of this compound is scaled up using similar synthetic routes but optimized for large-scale synthesis. This involves:
Batch Reactors: Utilizing batch reactors for the coupling reactions.
Continuous Flow Systems: Implementing continuous flow systems to enhance reaction efficiency and product consistency.
Quality Control: Rigorous quality control measures, including high-performance liquid chromatography (HPLC) and mass spectrometry, are employed to ensure the purity and consistency of the final product.
Analyse Des Réactions Chimiques
Types of Reactions
2-Chloro-6-amino-9-(2’,3’,5’-tri-O-acetyl-beta-D-ribofuranosyl)purine undergoes several types of chemical reactions, including:
Substitution Reactions: The chlorine atom can be substituted with various nucleophiles, such as amines or thiols, under appropriate conditions.
Deprotection Reactions: The acetyl groups can be removed under basic conditions to yield the free nucleoside.
Oxidation and Reduction: The purine ring can undergo oxidation and reduction reactions, although these are less common.
Common Reagents and Conditions
Nucleophilic Substitution: Reagents such as sodium azide or primary amines in polar aprotic solvents.
Deprotection: Sodium methoxide in methanol is commonly used for deacetylation.
Oxidation: Potassium permanganate or other strong oxidizing agents.
Reduction: Sodium borohydride or other reducing agents.
Major Products
Substituted Nucleosides: Depending on the nucleophile used, various substituted nucleosides can be obtained.
Free Nucleoside: Deacetylation yields the free nucleoside, which can be further modified or used in biological assays.
Applications De Recherche Scientifique
Medicinal Chemistry Applications
1. Anticancer Activity
Research indicates that derivatives of 2-chloro-6-amino purines exhibit antiproliferative effects against various cancer cell lines. For instance, studies have shown that compounds related to this structure can inhibit the growth of breast cancer (SK-BR-3 and MCF-7) and colon cancer (HCT116) cells, demonstrating moderate cytostatic activity when tested in vitro at concentrations around 10 µM .
2. Enzyme Substrates
The compound is also a substrate for specific enzymes such as purine nucleoside phosphorylase (PNP). It has been utilized to synthesize various C-6 substituted purine derivatives through cross-coupling reactions with organozinc reagents. This method has shown promise in producing compounds with potential therapeutic applications .
Synthesis and Structural Characterization
The synthesis of 2-chloro-6-amino-9-(2',3',5'-tri-O-acetyl-beta-D-ribofuranosyl)purine involves several steps:
- The initial chlorination of ribofuranose derivatives leads to the formation of the triacetate.
- Subsequent reactions with chlorinated precursors yield the desired purine nucleosides.
This synthetic pathway has been optimized for yield and purity, allowing for further investigations into biological activities .
Case Studies and Research Findings
Case Study 1: Antiproliferative Effects
In a study evaluating the antiproliferative effects of various purine derivatives, including 2-chloro-6-amino compounds, researchers found that these compounds could significantly inhibit cell growth in certain cancer lines. The mechanism of action appears to be linked to their ability to interfere with nucleotide metabolism within the cells .
Case Study 2: Enzymatic Reactions
Another study focused on the substrate activity of 2-chloro derivatives with Escherichia coli PNP. The findings indicated that these compounds could be effectively phosphorylated, leading to the formation of new nucleoside analogs that may have enhanced biological activity .
Mécanisme D'action
The mechanism of action of 2-Chloro-6-amino-9-(2’,3’,5’-tri-O-acetyl-beta-D-ribofuranosyl)purine involves its incorporation into nucleic acids, where it can interfere with normal cellular processes. This compound targets viral polymerases and other enzymes involved in nucleic acid synthesis, thereby inhibiting viral replication. In cancer cells, it can induce apoptosis by disrupting DNA synthesis and repair pathways.
Comparaison Avec Des Composés Similaires
Similar Compounds
2-Chloro-adenosine: Another nucleoside analog with similar antiviral properties.
6-Amino-2-chloropurine: Shares structural similarities but lacks the ribofuranosyl moiety.
2’,3’,5’-Tri-O-acetyladenosine: Similar in structure but without the chlorine substitution.
Uniqueness
2-Chloro-6-amino-9-(2’,3’,5’-tri-O-acetyl-beta-D-ribofuranosyl)purine is unique due to its specific combination of a chlorinated purine base and an acetylated ribofuranosyl sugar. This combination enhances its stability and bioavailability, making it particularly effective in antiviral and anticancer applications.
Activité Biologique
2-Chloro-6-amino-9-(2',3',5'-tri-O-acetyl-beta-D-ribofuranosyl)purine is a purine derivative that has garnered attention for its potential biological activities, particularly in the fields of oncology and virology. This article aims to provide a comprehensive overview of its biological activity, including synthesis, mechanisms of action, cytotoxicity, and case studies that illustrate its therapeutic potential.
- Molecular Formula: C16H18ClN5O7
- Molecular Weight: 427.80 g/mol
- CAS Number: 79999-39-6
- Purity: >95% (HPLC) .
Synthesis
The compound can be synthesized through various methods, including transglycosylation reactions that yield purine arabinosides with chiral amino acid amides at the C6 position. This method has been explored to enhance solubility and biological activity .
The biological activity of this compound is primarily attributed to its role as an inhibitor of purine biosynthesis. It selectively targets enzymes involved in the de novo synthesis pathway, particularly glycinamide ribonucleotide formyltransferase (GARFTase), which is crucial for tumor cell proliferation .
Antitumor Activity
Research indicates that this compound exhibits significant cytotoxic effects against various cancer cell lines. For instance:
- Cytotoxicity Testing : In vitro studies have demonstrated that the compound shows potent inhibitory effects on KB and IGROV1 human tumor cells, with IC50 values indicating high efficacy ( ).
- In Vivo Studies : In SCID mice with KB tumors, the compound displayed a remarkable ability to induce tumor regression, achieving complete remissions in several cases ( ).
Antiviral Activity
There are indications that derivatives of this compound may also possess antiviral properties. For example, related purine derivatives have shown activity against herpes simplex virus type 1 (HSV-1), suggesting potential for broader antiviral applications .
Case Studies
Study | Cell Line | IC50 (nM) | Outcome |
---|---|---|---|
Study 1 | KB Cells | 0.55 | High cytotoxicity observed |
Study 2 | IGROV1 Cells | 0.97 | Effective inhibition of cell growth |
Study 3 | Murine Mammary Carcinoma (4T1) | <30 | Significant cytotoxic effects noted |
Q & A
Q. Basic: What synthetic strategies are used to introduce chloro and amino groups at the C-2 and C-6 positions of ribofuranosylpurine derivatives?
Methodological Answer:
The synthesis involves sequential functionalization of the purine ring and ribofuranosyl backbone. Key steps include:
- Selective Acetylation : Protect ribose hydroxyls using acetic anhydride, triethylamine, and 4-(dimethylamino)pyridine (DMAP) in acetonitrile (93% yield) .
- Halogenation : Introduce chlorine at C-6 via nucleophilic substitution or direct halogenation of guanosine derivatives. For example, 6-chloro intermediates are synthesized using thiourea or iodine in controlled conditions .
- Amination : Replace C-6 chlorine with amino groups using ammonia or substituted amines. Thiourea in ethanol under reflux (3 hours, 90°C) is effective for thiolactam formation, which can be further modified .
- Cross-Coupling at C-2 : Pd-catalyzed Stille couplings (e.g., tributyl(vinyl)tin with Pd(CH₃CN)₂Cl₂ in DMF) introduce vinyl or aryl groups at C-2 .
Q. Basic: How is the structure of this compound confirmed using spectroscopic methods?
Methodological Answer:
Critical spectroscopic markers include:
- ¹H NMR :
- N-1 Proton : A broad singlet at ~11.41 ppm (CDCl₃) confirms thiolactam formation after C-6 substitution .
- Ribose Protons : Multiplets between δ 4.34–6.16 ppm (e.g., H-1' at δ 6.16, J = 4.3 Hz) validate the ribofuranosyl structure .
- ¹³C NMR :
- Thiocarbonyl Peak : A signal at ~178.2 ppm (C-6) distinguishes thiolactam from chloro precursors (C-6 Cl at ~159.5 ppm) .
- HRMS : Exact mass matching (e.g., [M+H]⁺ calculated 311.0814, observed 311.0810) confirms molecular integrity .
Table 1: Key NMR Data for Structural Confirmation
Position | ¹H NMR (δ, ppm) | ¹³C NMR (δ, ppm) | Functional Group |
---|---|---|---|
N-1 (Thiolactam) | 11.41 (br s) | - | NH in thiolactam |
C-6 (Thiocarbonyl) | - | 178.2 | C=S |
Ribose H-1' | 6.16 (d, J=4.3 Hz) | 87.1 | Anomeric carbon |
Q. Advanced: What side reactions occur during Pd-catalyzed cross-coupling, and how are they mitigated?
Methodological Answer:
Common issues include:
- Pd Residues : Residual palladium can catalyze undesired reactions. Mitigation involves filtration through Celite and washing with hexanes .
- Vinyl Group Isomerization : Prolonged heating (>4 hours at 90°C) may cause E/Z isomerization. Optimize reaction time and monitor via ¹H NMR (vinyl protons at δ 6.70–6.90 ppm) .
- Tin Byproducts : Excess tributyltin reagents are removed by hexane washes or silica gel chromatography .
Q. Advanced: How do protecting groups influence reactivity in ribofuranosylpurine synthesis?
Methodological Answer:
- Acetyl Groups : Provide stability during halogenation and cross-coupling but require deprotection (e.g., methanolic NH₃) post-functionalization .
- Benzoyl vs. Acetyl : Benzoyl groups offer higher thermal stability but complicate deprotection. Acetyl groups are preferred for stepwise synthesis due to milder cleavage conditions .
Q. Basic: What deprotection methods remove acetyl groups without damaging the purine base?
Methodological Answer:
- Methanolic Ammonia : Saturated NH₃/MeOH (overnight, room temperature) cleaves acetyl groups efficiently (90% yield). Monitor via TLC (Rf shift) or loss of acetyl signals in ¹H NMR (δ 2.06–2.17 ppm) .
- K₂CO₃/MeOH : Mild base conditions (8 hours, room temperature) selectively deprotect without purine degradation .
Q. Advanced: How are conflicting NMR data resolved during structural elucidation?
Methodological Answer:
- 2D NMR (COSY, HSQC) : Resolve overlapping signals by correlating ¹H-¹H and ¹H-¹³C couplings. For example, HSQC confirms ribose H-1' coupling to C-1' (δ 87.1 ppm) .
- Variable Temperature NMR : Suppress broad NH signals (e.g., N-1 proton) by cooling to 0°C .
- Comparative Analysis : Contrast spectra with known intermediates (e.g., 6-Cl vs. 6-SH derivatives) to assign ambiguous peaks .
Q. Advanced: What strategies optimize yield in C-6 substitution reactions?
Methodological Answer:
- Solvent Choice : Polar aprotic solvents (DMF, ethanol) enhance nucleophilic substitution at C-6. Ethanol is optimal for thiourea-mediated thiolactam formation (82% yield) .
- Drying Agents : Pre-dry reactants with molecular sieves to prevent hydrolysis of Sn or Pd reagents .
- Stoichiometry : Use 5–10 equivalents of nucleophiles (e.g., thiourea) to drive reactions to completion .
Q. Basic: What purification techniques are effective for acetylated intermediates?
Methodological Answer:
Propriétés
IUPAC Name |
[(2R,3S,4S,5R)-3,4-diacetyloxy-5-(6-amino-2-chloropurin-9-yl)oxolan-2-yl]methyl acetate | |
---|---|---|
Source | PubChem | |
URL | https://pubchem.ncbi.nlm.nih.gov | |
Description | Data deposited in or computed by PubChem | |
InChI |
InChI=1S/C16H18ClN5O7/c1-6(23)26-4-9-11(27-7(2)24)12(28-8(3)25)15(29-9)22-5-19-10-13(18)20-16(17)21-14(10)22/h5,9,11-12,15H,4H2,1-3H3,(H2,18,20,21)/t9-,11+,12+,15-/m1/s1 | |
Source | PubChem | |
URL | https://pubchem.ncbi.nlm.nih.gov | |
Description | Data deposited in or computed by PubChem | |
InChI Key |
IMTFOTCIQPKKSP-CKRXIKOQSA-N | |
Source | PubChem | |
URL | https://pubchem.ncbi.nlm.nih.gov | |
Description | Data deposited in or computed by PubChem | |
Canonical SMILES |
CC(=O)OCC1C(C(C(O1)N2C=NC3=C(N=C(N=C32)Cl)N)OC(=O)C)OC(=O)C | |
Source | PubChem | |
URL | https://pubchem.ncbi.nlm.nih.gov | |
Description | Data deposited in or computed by PubChem | |
Isomeric SMILES |
CC(=O)OC[C@@H]1[C@@H]([C@@H]([C@@H](O1)N2C=NC3=C(N=C(N=C32)Cl)N)OC(=O)C)OC(=O)C | |
Source | PubChem | |
URL | https://pubchem.ncbi.nlm.nih.gov | |
Description | Data deposited in or computed by PubChem | |
Molecular Formula |
C16H18ClN5O7 | |
Source | PubChem | |
URL | https://pubchem.ncbi.nlm.nih.gov | |
Description | Data deposited in or computed by PubChem | |
Molecular Weight |
427.8 g/mol | |
Source | PubChem | |
URL | https://pubchem.ncbi.nlm.nih.gov | |
Description | Data deposited in or computed by PubChem | |
Avertissement et informations sur les produits de recherche in vitro
Veuillez noter que tous les articles et informations sur les produits présentés sur BenchChem sont destinés uniquement à des fins informatives. Les produits disponibles à l'achat sur BenchChem sont spécifiquement conçus pour des études in vitro, qui sont réalisées en dehors des organismes vivants. Les études in vitro, dérivées du terme latin "in verre", impliquent des expériences réalisées dans des environnements de laboratoire contrôlés à l'aide de cellules ou de tissus. Il est important de noter que ces produits ne sont pas classés comme médicaments et n'ont pas reçu l'approbation de la FDA pour la prévention, le traitement ou la guérison de toute condition médicale, affection ou maladie. Nous devons souligner que toute forme d'introduction corporelle de ces produits chez les humains ou les animaux est strictement interdite par la loi. Il est essentiel de respecter ces directives pour assurer la conformité aux normes légales et éthiques en matière de recherche et d'expérimentation.