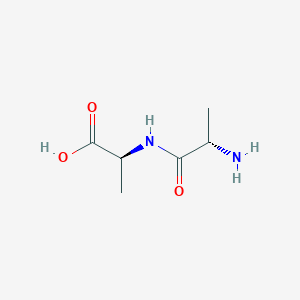
L-Alanyl-L-alanine
Vue d'ensemble
Description
L-Alanyl-L-alanine is a dipeptide composed of two L-alanine molecules linked by a peptide bond It is a simple dipeptide that plays a significant role in various biochemical processes
Applications De Recherche Scientifique
L-Alanyl-L-alanine has several applications in scientific research:
Chemistry: Used as a model compound to study peptide bond formation and stability.
Biology: Investigated for its role in protein metabolism and as a substrate for peptidases.
Medicine: Explored for its potential in drug delivery systems due to its biocompatibility.
Industry: Utilized in the production of biodegradable polymers and as a building block for more complex peptides.
Mécanisme D'action
Target of Action
L-Alanyl-L-alanine is a dipeptide consisting of two L-alanine units . It is involved in various biochemical processes, including metabolism, cell proliferation, protein synthesis, and degradation . It plays a significant role in immune response and glucose metabolism .
Mode of Action
This compound is produced from pyruvate by transamination . It interacts with its targets, leading to changes in cellular metabolism and proliferation, as well as immune regulation .
Biochemical Pathways
This compound is involved in several biochemical pathways. It is produced from pyruvate, the final product of the EMP pathway . During cell culture, this compound is broken down into L-glutamine, which is an essential nutrient for the cells .
Pharmacokinetics
It is known that this compound is stable in aqueous solution unlike l-glutamine which spontaneously degrades to form ammonia and pyrrolidine carboxylic acid . This stability makes it valuable in parenteral nutrition .
Result of Action
The action of this compound results in the production of L-glutamine, an essential nutrient for cells . This process is gradual, allowing cells to utilize the L-glutamine before it degrades into ammonia and pyrrolidine carboxylic acid . Ammonia can damage cells, so the use of this compound can help maintain cell health .
Action Environment
The action of this compound can be influenced by environmental factors. For example, its solubility and stability can be affected by temperature and pressure . In cell culture, the medium used can also impact the action of this compound .
Analyse Biochimique
Biochemical Properties
L-Alanyl-L-alanine interacts with several enzymes, proteins, and other biomolecules. One key enzyme it interacts with is Alanine dehydrogenase (AlaDH), which plays a crucial role in microbial carbon and nitrogen metabolism, spore formation, and photosynthesis . AlaDH can also be applied in the biosynthesis of L-alanine from cheap carbon sources, such as glucose .
Cellular Effects
This compound has substantial effects on various types of cells and cellular processes. For instance, it has been found that L-alanine and D-alanine can substantially elevate the titer of poly-γ-glutamic acid (γ-PGA), influencing cell function .
Molecular Mechanism
At the molecular level, this compound exerts its effects through binding interactions with biomolecules, enzyme inhibition or activation, and changes in gene expression. For example, AlaDH can catalyze the oxidation of alanine with NAD+ (NADP+) as coenzyme and the reduction of pyruvate with NADH (NADPH) as coenzyme .
Metabolic Pathways
This compound is involved in several metabolic pathways. It interacts with enzymes such as AlaDH and affects metabolic flux or metabolite levels .
Méthodes De Préparation
Synthetic Routes and Reaction Conditions: L-Alanyl-L-alanine can be synthesized through chemical and enzymatic methods. One common chemical synthesis involves the coupling of L-alanine with L-alanine methyl ester hydrochloride in the presence of a coupling agent such as dicyclohexylcarbodiimide (DCC) and a catalyst like 1-hydroxybenzotriazole (HOBt). The reaction is typically carried out in an organic solvent such as dimethylformamide (DMF) at room temperature.
Industrial Production Methods: Industrial production of this compound often employs enzymatic synthesis due to its higher specificity and yield. Enzymes such as amino acid ligases or peptidases are used to catalyze the formation of the dipeptide from L-alanine. This method is more environmentally friendly and can be scaled up for large-scale production.
Analyse Des Réactions Chimiques
Types of Reactions: L-Alanyl-L-alanine undergoes various chemical reactions, including hydrolysis, cyclization, and epimerization. Hydrolysis of the dipeptide results in the formation of two L-alanine molecules. Cyclization can lead to the formation of diketopiperazine, a cyclic dipeptide. Epimerization involves the conversion of one of the L-alanine residues to its D-form under certain conditions.
Common Reagents and Conditions:
Hydrolysis: Typically carried out in aqueous solutions under acidic or basic conditions.
Cyclization: Can occur under neutral or slightly acidic conditions.
Epimerization: Induced by exposure to strong acids or bases, or by irradiation with γ-rays.
Major Products:
Hydrolysis: L-alanine
Cyclization: Diketopiperazine
Epimerization: D-alanyl-L-alanine
Comparaison Avec Des Composés Similaires
- L-Alanyl-L-valine
- L-Valyl-L-alanine
- L-Alanyl-L-glutamine
L-Alanyl-L-alanine stands out due to its simplicity and versatility, making it a valuable compound for various applications in research and industry.
Activité Biologique
L-Alanyl-L-alanine (Ala-Ala) is a dipeptide composed of two L-alanine residues. It has garnered attention in various biological studies due to its significant roles in microbial metabolism, potential therapeutic applications, and its influence on cellular processes. This article explores the biological activity of this compound, focusing on its mechanisms of action, effects on different organisms, and relevant research findings.
This compound exhibits several biological activities primarily linked to its role as a substrate in metabolic pathways and its influence on cellular physiology:
- Metabolic Pathways : In Escherichia coli, Ala-Ala is metabolized to yield L-alanine, which plays a crucial role in protein synthesis and energy metabolism. The presence of Ala-Ala leads to the accumulation of L-alanine in the extracellular environment, indicating an active export mechanism that helps maintain intracellular balance .
- Inhibition of Pathogens : Research has shown that Ala-Ala can exhibit antimicrobial properties. For instance, studies have demonstrated that certain bacterial strains are more susceptible to Ala-Ala under conditions where their alanine export systems are compromised. This suggests that Ala-Ala may inhibit bacterial growth by disrupting amino acid homeostasis .
- Influence on Immune Response : In clinical settings, supplementation with L-alanyl-L-glutamine (a related compound) has been associated with improved immune responses in patients undergoing total parenteral nutrition (TPN). This effect is believed to stem from enhanced nitrogen balance and improved leukocyte function, which may also apply to this compound .
Table 1: Summary of Key Research Studies on this compound
Study | Organism | Key Findings | Methodology |
---|---|---|---|
Kim & Hori (2019) | E. coli | Identified the role of AlaE in regulating intracellular L-alanine levels; susceptibility tests showed lower MIC for mutants lacking alanine export systems | Susceptibility testing and genetic analysis |
Padia & Rao (1985) | Various | Reported antifungal activity of Ala-Ala; suggested potential as a therapeutic agent | Biological assays |
Strych et al. (2001) | E. coli | Mutant strains showed increased sensitivity to Ala-Ala; indicated the importance of alanine export mechanisms | Genetic mutagenesis and growth assays |
Fresenius Kabi Study (2004) | Human subjects | Improved infectious morbidity in patients receiving L-alanyl-L-glutamine-supplemented TPN compared to standard TPN | Randomized clinical trial |
Case Studies
- Microbial Sensitivity Testing : A study conducted by Kim and Hori evaluated the minimum inhibitory concentration (MIC) of Ala-Ala against various E. coli strains. The results indicated that strains deficient in alanine export mechanisms exhibited significantly lower MIC values, suggesting that the accumulation of intracellular L-alanine due to impaired export led to increased susceptibility to Ala-Ala .
- Clinical Application in TPN : In a randomized clinical trial involving patients with secondary peritonitis, those receiving L-alanyl-L-glutamine-enriched TPN showed a notable reduction in infectious morbidity compared to those on standard TPN. This highlights the potential benefits of amino acid supplementation in enhancing patient recovery post-surgery .
Propriétés
IUPAC Name |
(2S)-2-[[(2S)-2-aminopropanoyl]amino]propanoic acid | |
---|---|---|
Source | PubChem | |
URL | https://pubchem.ncbi.nlm.nih.gov | |
Description | Data deposited in or computed by PubChem | |
InChI |
InChI=1S/C6H12N2O3/c1-3(7)5(9)8-4(2)6(10)11/h3-4H,7H2,1-2H3,(H,8,9)(H,10,11)/t3-,4-/m0/s1 | |
Source | PubChem | |
URL | https://pubchem.ncbi.nlm.nih.gov | |
Description | Data deposited in or computed by PubChem | |
InChI Key |
DEFJQIDDEAULHB-IMJSIDKUSA-N | |
Source | PubChem | |
URL | https://pubchem.ncbi.nlm.nih.gov | |
Description | Data deposited in or computed by PubChem | |
Canonical SMILES |
CC(C(=O)NC(C)C(=O)O)N | |
Source | PubChem | |
URL | https://pubchem.ncbi.nlm.nih.gov | |
Description | Data deposited in or computed by PubChem | |
Isomeric SMILES |
C[C@@H](C(=O)N[C@@H](C)C(=O)O)N | |
Source | PubChem | |
URL | https://pubchem.ncbi.nlm.nih.gov | |
Description | Data deposited in or computed by PubChem | |
Molecular Formula |
C6H12N2O3 | |
Source | PubChem | |
URL | https://pubchem.ncbi.nlm.nih.gov | |
Description | Data deposited in or computed by PubChem | |
DSSTOX Substance ID |
DTXSID00173127 | |
Record name | L-Alanyl-L-alanine | |
Source | EPA DSSTox | |
URL | https://comptox.epa.gov/dashboard/DTXSID00173127 | |
Description | DSSTox provides a high quality public chemistry resource for supporting improved predictive toxicology. | |
Molecular Weight |
160.17 g/mol | |
Source | PubChem | |
URL | https://pubchem.ncbi.nlm.nih.gov | |
Description | Data deposited in or computed by PubChem | |
Physical Description |
Solid | |
Record name | Alanylalanine | |
Source | Human Metabolome Database (HMDB) | |
URL | http://www.hmdb.ca/metabolites/HMDB0028680 | |
Description | The Human Metabolome Database (HMDB) is a freely available electronic database containing detailed information about small molecule metabolites found in the human body. | |
Explanation | HMDB is offered to the public as a freely available resource. Use and re-distribution of the data, in whole or in part, for commercial purposes requires explicit permission of the authors and explicit acknowledgment of the source material (HMDB) and the original publication (see the HMDB citing page). We ask that users who download significant portions of the database cite the HMDB paper in any resulting publications. | |
CAS No. |
1948-31-8, 1644661-10-8 | |
Record name | L-Alanyl-L-alanine | |
Source | CAS Common Chemistry | |
URL | https://commonchemistry.cas.org/detail?cas_rn=1948-31-8 | |
Description | CAS Common Chemistry is an open community resource for accessing chemical information. Nearly 500,000 chemical substances from CAS REGISTRY cover areas of community interest, including common and frequently regulated chemicals, and those relevant to high school and undergraduate chemistry classes. This chemical information, curated by our expert scientists, is provided in alignment with our mission as a division of the American Chemical Society. | |
Explanation | The data from CAS Common Chemistry is provided under a CC-BY-NC 4.0 license, unless otherwise stated. | |
Record name | Dialanine | |
Source | ChemIDplus | |
URL | https://pubchem.ncbi.nlm.nih.gov/substance/?source=chemidplus&sourceid=0001948318 | |
Description | ChemIDplus is a free, web search system that provides access to the structure and nomenclature authority files used for the identification of chemical substances cited in National Library of Medicine (NLM) databases, including the TOXNET system. | |
Record name | D-Alanyl-D-alanine, (+/-)- | |
Source | ChemIDplus | |
URL | https://pubchem.ncbi.nlm.nih.gov/substance/?source=chemidplus&sourceid=1644661108 | |
Description | ChemIDplus is a free, web search system that provides access to the structure and nomenclature authority files used for the identification of chemical substances cited in National Library of Medicine (NLM) databases, including the TOXNET system. | |
Record name | L-Alanyl-L-alanine | |
Source | EPA DSSTox | |
URL | https://comptox.epa.gov/dashboard/DTXSID00173127 | |
Description | DSSTox provides a high quality public chemistry resource for supporting improved predictive toxicology. | |
Record name | N-L-alanyl-L-alanine | |
Source | European Chemicals Agency (ECHA) | |
URL | https://echa.europa.eu/substance-information/-/substanceinfo/100.016.138 | |
Description | The European Chemicals Agency (ECHA) is an agency of the European Union which is the driving force among regulatory authorities in implementing the EU's groundbreaking chemicals legislation for the benefit of human health and the environment as well as for innovation and competitiveness. | |
Explanation | Use of the information, documents and data from the ECHA website is subject to the terms and conditions of this Legal Notice, and subject to other binding limitations provided for under applicable law, the information, documents and data made available on the ECHA website may be reproduced, distributed and/or used, totally or in part, for non-commercial purposes provided that ECHA is acknowledged as the source: "Source: European Chemicals Agency, http://echa.europa.eu/". Such acknowledgement must be included in each copy of the material. ECHA permits and encourages organisations and individuals to create links to the ECHA website under the following cumulative conditions: Links can only be made to webpages that provide a link to the Legal Notice page. | |
Record name | D-ALANYL-D-ALANINE, (±)- | |
Source | FDA Global Substance Registration System (GSRS) | |
URL | https://gsrs.ncats.nih.gov/ginas/app/beta/substances/37AOH3N58S | |
Description | The FDA Global Substance Registration System (GSRS) enables the efficient and accurate exchange of information on what substances are in regulated products. Instead of relying on names, which vary across regulatory domains, countries, and regions, the GSRS knowledge base makes it possible for substances to be defined by standardized, scientific descriptions. | |
Explanation | Unless otherwise noted, the contents of the FDA website (www.fda.gov), both text and graphics, are not copyrighted. They are in the public domain and may be republished, reprinted and otherwise used freely by anyone without the need to obtain permission from FDA. Credit to the U.S. Food and Drug Administration as the source is appreciated but not required. | |
Record name | DIALANINE | |
Source | FDA Global Substance Registration System (GSRS) | |
URL | https://gsrs.ncats.nih.gov/ginas/app/beta/substances/MY4X7L3WAM | |
Description | The FDA Global Substance Registration System (GSRS) enables the efficient and accurate exchange of information on what substances are in regulated products. Instead of relying on names, which vary across regulatory domains, countries, and regions, the GSRS knowledge base makes it possible for substances to be defined by standardized, scientific descriptions. | |
Explanation | Unless otherwise noted, the contents of the FDA website (www.fda.gov), both text and graphics, are not copyrighted. They are in the public domain and may be republished, reprinted and otherwise used freely by anyone without the need to obtain permission from FDA. Credit to the U.S. Food and Drug Administration as the source is appreciated but not required. | |
Record name | Alanylalanine | |
Source | Human Metabolome Database (HMDB) | |
URL | http://www.hmdb.ca/metabolites/HMDB0028680 | |
Description | The Human Metabolome Database (HMDB) is a freely available electronic database containing detailed information about small molecule metabolites found in the human body. | |
Explanation | HMDB is offered to the public as a freely available resource. Use and re-distribution of the data, in whole or in part, for commercial purposes requires explicit permission of the authors and explicit acknowledgment of the source material (HMDB) and the original publication (see the HMDB citing page). We ask that users who download significant portions of the database cite the HMDB paper in any resulting publications. | |
Retrosynthesis Analysis
AI-Powered Synthesis Planning: Our tool employs the Template_relevance Pistachio, Template_relevance Bkms_metabolic, Template_relevance Pistachio_ringbreaker, Template_relevance Reaxys, Template_relevance Reaxys_biocatalysis model, leveraging a vast database of chemical reactions to predict feasible synthetic routes.
One-Step Synthesis Focus: Specifically designed for one-step synthesis, it provides concise and direct routes for your target compounds, streamlining the synthesis process.
Accurate Predictions: Utilizing the extensive PISTACHIO, BKMS_METABOLIC, PISTACHIO_RINGBREAKER, REAXYS, REAXYS_BIOCATALYSIS database, our tool offers high-accuracy predictions, reflecting the latest in chemical research and data.
Strategy Settings
Precursor scoring | Relevance Heuristic |
---|---|
Min. plausibility | 0.01 |
Model | Template_relevance |
Template Set | Pistachio/Bkms_metabolic/Pistachio_ringbreaker/Reaxys/Reaxys_biocatalysis |
Top-N result to add to graph | 6 |
Feasible Synthetic Routes
Q1: What is the molecular formula and weight of L-alanyl-L-alanine?
A1: The molecular formula of this compound is C6H12N2O3, and its molecular weight is 160.17 g/mol.
Q2: How does the conformation of this compound change depending on pH?
A2: this compound exhibits different conformations depending on the pH of the solution, influenced by its charge state. [, ] While the cationic (AA+) and zwitterionic (AAZW) forms exhibit similar main-chain conformations, the anionic form (AA-) adopts an additional conformation in aqueous solution, characterized by a different backbone psi angle and rotation around the NH2 group. []
Q3: How do spectroscopic techniques like NMR and ROA contribute to understanding the structure and conformation of this compound?
A3: Nuclear Magnetic Resonance (NMR) spectroscopy provides valuable information about the local conformation of this compound through chemical shifts and spin-spin coupling constants. [, ] These parameters, particularly the coupling constants, are highly sensitive to dihedral angles and can help determine the three-dimensional arrangement of atoms within the molecule. [] Raman Optical Activity (ROA), on the other hand, offers insights into the chiral properties and subtle conformational changes in Ala-Ala, especially when combined with theoretical calculations and isotopic labeling. [, ]
Q4: How does the conformation of this compound influence its interaction with water molecules?
A4: The conformation of this compound directly influences its hydrogen bonding interactions with water molecules. Studies employing solution- and solid-state NMR have shown that solvation leads to changes in hydrogen bonding patterns compared to the crystalline state. [] These changes in hydrogen bonding, driven by the interaction with water, contribute to the different conformations observed for this compound in solution. []
Q5: How does the dipeptide approximation hold up when studying this compound, and what are its limitations?
A5: While the dipeptide approximation, which assumes independent residue behavior, is commonly used in peptide conformational analysis, studies on N-formyl-L-alanyl-L-alanine amide (ALA-ALA) highlight its limitations. [, ] The conformation of a residue within the tripeptide is significantly influenced by the conformational state of its neighboring residue, showcasing the importance of considering interresidue interactions for accurate structural predictions. [, ]
Q6: How does this compound interact with porphyrins in aqueous solution?
A6: this compound, along with its derivatives like L-lysyl-L-alanyl-L-alanine, interacts with porphyrins like meso-tetrakis(4-sulfonatophenyl)porphine (TPPS) through electrostatic interactions. [, ] These interactions can induce conformational changes in the peptide, shifting from a polyproline II (PPII) helix to a more extended structure resembling a β-sheet. [, ] The presence of metal ions and axial ligands in metalloporphyrins further modulates the interaction and influences the peptide conformation. []
Q7: Can this compound be used as a substrate to study enzyme activity?
A7: Yes, this compound serves as a specific substrate for enzymes like dipeptidyl peptidase IV and elastase. [, , ] Researchers can monitor the hydrolysis of this compound or its derivatives, such as N-acetyl-L-alanyl-L-alanyl-L-alanine methyl ester, to determine enzyme activity and study their kinetic parameters. [, , , ] This approach allows for characterizing enzyme specificity and investigating potential inhibitors.
Q8: How do computational methods contribute to understanding the properties and behavior of this compound?
A8: Computational chemistry, particularly density functional theory (DFT) calculations, plays a significant role in studying this compound. [, , ] DFT enables researchers to predict various molecular properties, including geometries, vibrational frequencies, NMR chemical shifts, and chemical shielding tensors, offering deeper insights into its structure, dynamics, and interactions with the environment. [, , ] These calculations aid in interpreting experimental data and provide a theoretical framework for understanding the behavior of this compound in different chemical environments.
Q9: What are the advantages of using stable isotopes in combination with computational methods to study this compound?
A9: Combining stable isotope labeling with computational methods, particularly DFT calculations, provides a powerful approach to study this compound. [, ] Isotopic labeling, such as using 13C and 15N, introduces distinct NMR-active nuclei, facilitating the assignment of vibrational modes and the accurate calculation of NMR parameters like chemical shifts and coupling constants. [, ] This approach allows for a more reliable comparison between experimental and theoretical data, leading to a more comprehensive understanding of the structure and dynamics of this compound.
Q10: How can molecular dynamics simulations contribute to the study of this compound in solution?
A10: Molecular dynamics simulations allow researchers to study the dynamic behavior of this compound in solution. [] These simulations can model the interactions between this compound and water molecules, providing insights into its solvation shell, conformational preferences, and dynamic changes over time. [] This information complements experimental data obtained from techniques like NMR and ROA, contributing to a more complete understanding of the dipeptide's behavior in a biologically relevant environment.
Avertissement et informations sur les produits de recherche in vitro
Veuillez noter que tous les articles et informations sur les produits présentés sur BenchChem sont destinés uniquement à des fins informatives. Les produits disponibles à l'achat sur BenchChem sont spécifiquement conçus pour des études in vitro, qui sont réalisées en dehors des organismes vivants. Les études in vitro, dérivées du terme latin "in verre", impliquent des expériences réalisées dans des environnements de laboratoire contrôlés à l'aide de cellules ou de tissus. Il est important de noter que ces produits ne sont pas classés comme médicaments et n'ont pas reçu l'approbation de la FDA pour la prévention, le traitement ou la guérison de toute condition médicale, affection ou maladie. Nous devons souligner que toute forme d'introduction corporelle de ces produits chez les humains ou les animaux est strictement interdite par la loi. Il est essentiel de respecter ces directives pour assurer la conformité aux normes légales et éthiques en matière de recherche et d'expérimentation.