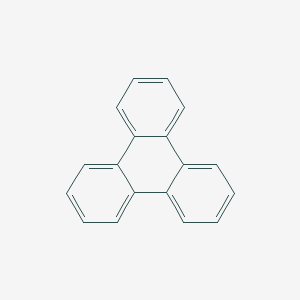
Triphénylène
Vue d'ensemble
Description
Triphenylene is a polycyclic aromatic hydrocarbon (PAH) compound that has been studied extensively due to its unique properties. It is an aromatic hydrocarbon composed of three phenyl rings connected by two meta-linked benzene rings. Triphenylene is a colorless solid that is insoluble in water and has a molecular weight of 202.29 g/mol. It is a highly stable compound that is resistant to oxidation and has a low volatility. Triphenylene has been used in a variety of applications, including in the synthesis of other compounds, in scientific research, and in laboratory experiments.
Applications De Recherche Scientifique
Matériau d'anode pour les batteries métal-ion
Le triphénylène a été théoriquement prédit comme un excellent matériau d'anode pour les batteries métal-ion, en particulier sous sa forme de graphdiyne phosphoré (P-TpG). Des simulations approfondies de la théorie de la fonctionnelle de la densité (DFT) suggèrent que les monocouches de P-TpG peuvent offrir des capacités de stockage ultra-élevées pour les ions Li, Na, K, Mg et Ca. Cela pourrait conduire au développement de batteries métal-ion avancées avec des capacités de charge remarquables et des taux de charge/décharge optimaux .
Comportement à haute pression et supraconductivité potentielle
Des études combinant des mesures expérimentales et des analyses computationnelles ont exploré le comportement à haute pression du this compound. La recherche indique que le this compound reste stable sous des pressions élevées sans transitions structurales. Des calculs théoriques suggèrent que sous des pressions extrêmes, le this compound pourrait devenir métallique, ce qui alimente les spéculations sur la réalisation potentielle de la supraconductivité à haute pression .
Propriétés de transport de charge
Les propriétés de transport de charge du this compound présentent un intérêt significatif dans la recherche scientifique. Des études théoriques se sont concentrées sur la compréhension de l'élément de la matrice de transfert de charge et du taux de transfert de charge, qui sont cruciaux pour le développement de dispositifs électroniques organiques .
Mécanisme D'action
Target of Action
Triphenylene is a flat polycyclic aromatic hydrocarbon (PAH) that consists of four fused benzene rings . It has delocalized 18-π-electron systems based on a planar structure
Mode of Action
Triphenylene’s mode of action is primarily through its interaction with other molecules via π-π stacking due to its planar structure and delocalized π-electron systems . This allows it to interact with a variety of biological and chemical systems.
Biochemical Pathways
It has been suggested that triphenylene may be involved in the high-temperature synthesis of pahs as detected in carbonaceous meteorites and proposed to exist in the interstellar medium (ism) . A study has presented evidence of a barrier-less pathway leading to a prototype tetracyclic PAH - triphenylene - via an unconventional Hydrogen Abstraction – Vinylacetylene Addition (HAVA) mechanism operational at temperatures as low as 10 K .
Pharmacokinetics
Its physical properties such as its density (1308 g/cm^3), melting point (198 °C), and boiling point (438 °C) suggest that it may have low bioavailability due to its high stability and low solubility .
Result of Action
Due to its planar structure and delocalized π-electron systems, it has attracted attention as the core of discotic mesogen in liquid crystalline materials .
Action Environment
The action of triphenylene can be influenced by environmental factors such as temperature and pressure. For instance, the HAVA mechanism that leads to the formation of triphenylene is operational at temperatures as low as 10 K . Furthermore, its physical properties such as its high melting and boiling points suggest that it is stable under a wide range of environmental conditions .
Safety and Hazards
Triphenylene causes serious eye damage . It is very toxic to aquatic life with long-lasting effects . It is advised to avoid dust formation, breathing mist, gas or vapors, and contact with skin and eyes . Protective equipment such as gloves and eye protection should be worn when handling triphenylene .
Orientations Futures
Triphenylene-based conductive MOFs are emerging to provide additional possibilities for multifunctional electronic devices . The 2D c-MOFs have displayed great potential for multiple high-performance (opto)electronic, magnetic, and energy devices . The study of amorphous CPs (aCPs) over the past decade has raised fundamental questions about the rational design of amorphous materials, and their potential applications in areas such as catalysis and biomedicine are promising .
Analyse Biochimique
Biochemical Properties
It is known that Triphenylene can participate in various chemical reactions, particularly those involving aromatic compounds
Temporal Effects in Laboratory Settings
The stability and degradation of Triphenylene in laboratory settings have not been extensively studied. It is known that Triphenylene can be synthesized in the gas phase , suggesting that it may be stable under certain conditions. Long-term effects on cellular function in in vitro or in vivo studies have not been reported.
Propriétés
IUPAC Name |
triphenylene | |
---|---|---|
Source | PubChem | |
URL | https://pubchem.ncbi.nlm.nih.gov | |
Description | Data deposited in or computed by PubChem | |
InChI |
InChI=1S/C18H12/c1-2-8-14-13(7-1)15-9-3-4-11-17(15)18-12-6-5-10-16(14)18/h1-12H | |
Source | PubChem | |
URL | https://pubchem.ncbi.nlm.nih.gov | |
Description | Data deposited in or computed by PubChem | |
InChI Key |
SLGBZMMZGDRARJ-UHFFFAOYSA-N | |
Source | PubChem | |
URL | https://pubchem.ncbi.nlm.nih.gov | |
Description | Data deposited in or computed by PubChem | |
Canonical SMILES |
C1=CC=C2C(=C1)C3=CC=CC=C3C4=CC=CC=C24 | |
Source | PubChem | |
URL | https://pubchem.ncbi.nlm.nih.gov | |
Description | Data deposited in or computed by PubChem | |
Molecular Formula |
C18H12 | |
Source | PubChem | |
URL | https://pubchem.ncbi.nlm.nih.gov | |
Description | Data deposited in or computed by PubChem | |
Related CAS |
53814-75-8 | |
Record name | Poly(triphenylene) | |
Source | CAS Common Chemistry | |
URL | https://commonchemistry.cas.org/detail?cas_rn=53814-75-8 | |
Description | CAS Common Chemistry is an open community resource for accessing chemical information. Nearly 500,000 chemical substances from CAS REGISTRY cover areas of community interest, including common and frequently regulated chemicals, and those relevant to high school and undergraduate chemistry classes. This chemical information, curated by our expert scientists, is provided in alignment with our mission as a division of the American Chemical Society. | |
Explanation | The data from CAS Common Chemistry is provided under a CC-BY-NC 4.0 license, unless otherwise stated. | |
DSSTOX Substance ID |
DTXSID9059757 | |
Record name | Triphenylene | |
Source | EPA DSSTox | |
URL | https://comptox.epa.gov/dashboard/DTXSID9059757 | |
Description | DSSTox provides a high quality public chemistry resource for supporting improved predictive toxicology. | |
Molecular Weight |
228.3 g/mol | |
Source | PubChem | |
URL | https://pubchem.ncbi.nlm.nih.gov | |
Description | Data deposited in or computed by PubChem | |
Physical Description |
Light yellow powder, insoluble in water; [MSDSonline] | |
Record name | Triphenylene | |
Source | Haz-Map, Information on Hazardous Chemicals and Occupational Diseases | |
URL | https://haz-map.com/Agents/7868 | |
Description | Haz-Map® is an occupational health database designed for health and safety professionals and for consumers seeking information about the adverse effects of workplace exposures to chemical and biological agents. | |
Explanation | Copyright (c) 2022 Haz-Map(R). All rights reserved. Unless otherwise indicated, all materials from Haz-Map are copyrighted by Haz-Map(R). No part of these materials, either text or image may be used for any purpose other than for personal use. Therefore, reproduction, modification, storage in a retrieval system or retransmission, in any form or by any means, electronic, mechanical or otherwise, for reasons other than personal use, is strictly prohibited without prior written permission. | |
CAS RN |
217-59-4 | |
Record name | Triphenylene | |
Source | CAS Common Chemistry | |
URL | https://commonchemistry.cas.org/detail?cas_rn=217-59-4 | |
Description | CAS Common Chemistry is an open community resource for accessing chemical information. Nearly 500,000 chemical substances from CAS REGISTRY cover areas of community interest, including common and frequently regulated chemicals, and those relevant to high school and undergraduate chemistry classes. This chemical information, curated by our expert scientists, is provided in alignment with our mission as a division of the American Chemical Society. | |
Explanation | The data from CAS Common Chemistry is provided under a CC-BY-NC 4.0 license, unless otherwise stated. | |
Record name | Triphenylene | |
Source | ChemIDplus | |
URL | https://pubchem.ncbi.nlm.nih.gov/substance/?source=chemidplus&sourceid=0000217594 | |
Description | ChemIDplus is a free, web search system that provides access to the structure and nomenclature authority files used for the identification of chemical substances cited in National Library of Medicine (NLM) databases, including the TOXNET system. | |
Record name | TRIPHENYLENE | |
Source | DTP/NCI | |
URL | https://dtp.cancer.gov/dtpstandard/servlet/dwindex?searchtype=NSC&outputformat=html&searchlist=57455 | |
Description | The NCI Development Therapeutics Program (DTP) provides services and resources to the academic and private-sector research communities worldwide to facilitate the discovery and development of new cancer therapeutic agents. | |
Explanation | Unless otherwise indicated, all text within NCI products is free of copyright and may be reused without our permission. Credit the National Cancer Institute as the source. | |
Record name | Triphenylene | |
Source | EPA DSSTox | |
URL | https://comptox.epa.gov/dashboard/DTXSID9059757 | |
Description | DSSTox provides a high quality public chemistry resource for supporting improved predictive toxicology. | |
Record name | Triphenylene | |
Source | European Chemicals Agency (ECHA) | |
URL | https://echa.europa.eu/substance-information/-/substanceinfo/100.005.385 | |
Description | The European Chemicals Agency (ECHA) is an agency of the European Union which is the driving force among regulatory authorities in implementing the EU's groundbreaking chemicals legislation for the benefit of human health and the environment as well as for innovation and competitiveness. | |
Explanation | Use of the information, documents and data from the ECHA website is subject to the terms and conditions of this Legal Notice, and subject to other binding limitations provided for under applicable law, the information, documents and data made available on the ECHA website may be reproduced, distributed and/or used, totally or in part, for non-commercial purposes provided that ECHA is acknowledged as the source: "Source: European Chemicals Agency, http://echa.europa.eu/". Such acknowledgement must be included in each copy of the material. ECHA permits and encourages organisations and individuals to create links to the ECHA website under the following cumulative conditions: Links can only be made to webpages that provide a link to the Legal Notice page. | |
Record name | TRIPHENYLENE | |
Source | FDA Global Substance Registration System (GSRS) | |
URL | https://gsrs.ncats.nih.gov/ginas/app/beta/substances/18WX3373I0 | |
Description | The FDA Global Substance Registration System (GSRS) enables the efficient and accurate exchange of information on what substances are in regulated products. Instead of relying on names, which vary across regulatory domains, countries, and regions, the GSRS knowledge base makes it possible for substances to be defined by standardized, scientific descriptions. | |
Explanation | Unless otherwise noted, the contents of the FDA website (www.fda.gov), both text and graphics, are not copyrighted. They are in the public domain and may be republished, reprinted and otherwise used freely by anyone without the need to obtain permission from FDA. Credit to the U.S. Food and Drug Administration as the source is appreciated but not required. | |
Synthesis routes and methods I
Procedure details
Synthesis routes and methods II
Procedure details
Synthesis routes and methods III
Procedure details
Retrosynthesis Analysis
AI-Powered Synthesis Planning: Our tool employs the Template_relevance Pistachio, Template_relevance Bkms_metabolic, Template_relevance Pistachio_ringbreaker, Template_relevance Reaxys, Template_relevance Reaxys_biocatalysis model, leveraging a vast database of chemical reactions to predict feasible synthetic routes.
One-Step Synthesis Focus: Specifically designed for one-step synthesis, it provides concise and direct routes for your target compounds, streamlining the synthesis process.
Accurate Predictions: Utilizing the extensive PISTACHIO, BKMS_METABOLIC, PISTACHIO_RINGBREAKER, REAXYS, REAXYS_BIOCATALYSIS database, our tool offers high-accuracy predictions, reflecting the latest in chemical research and data.
Strategy Settings
Precursor scoring | Relevance Heuristic |
---|---|
Min. plausibility | 0.01 |
Model | Template_relevance |
Template Set | Pistachio/Bkms_metabolic/Pistachio_ringbreaker/Reaxys/Reaxys_biocatalysis |
Top-N result to add to graph | 6 |
Feasible Synthetic Routes
Q & A
Q1: What is the molecular formula and weight of triphenylene?
A1: Triphenylene has the molecular formula C18H12 and a molecular weight of 228.29 g/mol.
Q2: How can the structure of triphenylene derivatives be confirmed?
A: The structure of triphenylene derivatives can be confirmed using various spectroscopic techniques. Common methods include proton nuclear magnetic resonance (1H NMR) spectroscopy, carbon-13 nuclear magnetic resonance (13C NMR) spectroscopy, infrared (IR) spectroscopy, and mass spectrometry (MS). [, , , , , , , , ]
Q3: What is the significance of the triphenylene core in material design?
A: The triphenylene core, with its planar aromatic structure, promotes strong π-π stacking interactions between molecules. This characteristic is crucial for the formation of ordered columnar structures in discotic liquid crystals. [, , , , , ]
Q4: What are the typical absorption and emission characteristics of triphenylenes?
A: Triphenylene derivatives commonly exhibit absorption bands in the UV region, often with a maximum around 280 nm. They can also display fluorescence, with emission wavelengths typically in the visible range. For instance, oligotriphenylene nanofibers show blue light emission. [, , , , ]
Q5: How does the introduction of different peripheral chains influence the properties of triphenylenes?
A: The choice of peripheral chains significantly impacts the properties of triphenylene derivatives. For instance, replacing alkoxy chains with ester chains or introducing alkyl chains can alter the mesophase behavior and stability. [, , , , , ]
Q6: What types of mesophases are commonly observed in triphenylene derivatives?
A: Triphenylene derivatives are well-known for their ability to exhibit discotic liquid crystal phases, particularly the columnar hexagonal (Colh) phase. [, , , , , , , , , ]
Q7: How do molecular symmetry and the presence of heteroatoms affect the mesomorphic behavior of triphenylenes?
A: Studies have shown that both molecular symmetry and the presence of heteroatoms play a crucial role in determining the mesomorphic behavior. For example, asymmetrical triphenylenes with mixed chains tend to have lower melting and clearing points compared to symmetrical counterparts. [, , ] The incorporation of oxygen atoms in ester chains can enhance the stability of the Colh mesophase. [] Incorporating sulfur can lead to the formation of helical mesophases, while alkyl substituents can suppress mesophase formation. [, ]
Q8: How does the length of the peripheral chains influence the mesophase behavior?
A: Longer alkoxy chains on triphenylenes generally lead to wider mesophase ranges and lower transition temperatures. [, ]
Q9: What is the fluorophilic effect in triphenylene discotic liquid crystals?
A: Introducing fluoroalkoxy chains into triphenylene discogens enhances the stability of the discotic columnar mesophase due to fluorophilic interactions. These interactions increase melting and clearing points compared to their non-fluorinated analogs. []
Q10: Why are triphenylene derivatives investigated for organic electronics?
A: The self-organization of triphenylenes into columnar structures enables efficient charge transport along the stacked aromatic cores, making them promising candidates for applications such as organic field-effect transistors (OFETs) and organic solar cells (OSCs). [, , , ]
Q11: How can the charge transport properties of triphenylenes be improved?
A: Charge transport can be enhanced by optimizing the molecular packing within the columnar structures, for example, by modifying the length or type of peripheral chains or introducing electron-withdrawing or donating groups. [, ]
Q12: What is the role of charge-transfer complexes in triphenylene-based materials?
A: Charge-transfer complexes formed between triphenylene derivatives and electron acceptors can induce or alter the mesomorphic behavior. For instance, triphenylene dimers that are not liquid crystalline in their pure form exhibit columnar mesophases upon complexation with 2,4,7-trinitrofluorenone (TNF). [, ]
Q13: What are common synthetic approaches to preparing triphenylene derivatives?
A: Triphenylenes can be synthesized via various routes, including oxidative cyclization reactions like the Scholl reaction, transition metal-catalyzed aryne cyclotrimerization, and nickel-mediated Yamamoto coupling of o-dibromoarenes. []
Q14: How can triphenylene derivatives be functionalized for specific applications?
A: Introducing functional groups to the triphenylene core or the peripheral chains can tailor the properties for specific applications. For example, incorporating reactive groups like epoxy groups enables the development of chemically amplified resists for lithography. []
Q15: What are the potential applications of triphenylene-based materials beyond organic electronics?
A: Research on triphenylene derivatives extends beyond electronics, exploring their potential in areas such as sensing applications and the synthesis of wire-assembled gold nanoparticles. [, , ]
Q16: How is computational chemistry used to study triphenylene derivatives?
A: Density functional theory (DFT) calculations are employed to investigate the electronic properties of triphenylene derivatives, such as their HOMO and LUMO energy levels, which are crucial for predicting their performance in organic electronic devices. [, , ]
Q17: What insights can be gained from computational studies on triphenylenes?
A: Computational modeling helps in understanding the relationship between molecular structure and properties, aiding in the design of new triphenylene derivatives with improved performance in various applications. []
Q18: How are range-separated functionals used in studying triphenylene-based polymers?
A: Range-separated functionals in time-dependent density functional theory (TDDFT) calculations are used to predict the luminescence energies and exciton localization in triphenylene-based polymers. This allows for a better understanding of the photophysical properties of these materials. []
Avertissement et informations sur les produits de recherche in vitro
Veuillez noter que tous les articles et informations sur les produits présentés sur BenchChem sont destinés uniquement à des fins informatives. Les produits disponibles à l'achat sur BenchChem sont spécifiquement conçus pour des études in vitro, qui sont réalisées en dehors des organismes vivants. Les études in vitro, dérivées du terme latin "in verre", impliquent des expériences réalisées dans des environnements de laboratoire contrôlés à l'aide de cellules ou de tissus. Il est important de noter que ces produits ne sont pas classés comme médicaments et n'ont pas reçu l'approbation de la FDA pour la prévention, le traitement ou la guérison de toute condition médicale, affection ou maladie. Nous devons souligner que toute forme d'introduction corporelle de ces produits chez les humains ou les animaux est strictement interdite par la loi. Il est essentiel de respecter ces directives pour assurer la conformité aux normes légales et éthiques en matière de recherche et d'expérimentation.